Synthesis of Key Ideas from the 2012 Workshop
Jump down to:
- 1. Understanding geologic time and rates is important, not only for geoscientists but for citizens of the planet.
- 2. Understanding geologic time is cognitively challenging.
- 3. While there has not been much research on best practices in teaching about time, there are promising strategies available.
- 4. Measuring student understanding of temporal concepts is challenging, but essential.
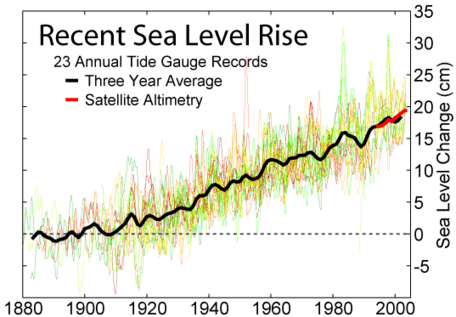
1. Understanding geologic time and rates is important, not only for geoscientists but for citizens of the planet. An understanding of the length of geologic time and the brevity of humans' existence is essential to understanding our place in the universe. Comparing the rate of change in Earth systems related to human activities and the rates associated with natural (non-anthropogenic) processes is fundamental to understanding how our actions affect the environment upon which we depend. Comprehending the depth of geologic time is a prerequisite for understanding:
- that the Earth has a long, complicated history, with more than 99.9% of it predating human existence;
- that the Earth is an evolving planet, changing over geologic time via both slow, long-term processes and sudden, short-term processes, as illustrated in this teaching activity on timescales of landscape evolution;
- that human activities have accelerated some of these processes well beyond their natural rates; and
- that Earth's history provides the context, as well as the data, needed to evaluate (a) the risks of a wide variety of geologic hazards and (b) the rates at which a wide variety of natural resources we use can be replenished.
As is the case with human history, understanding the history of the Earth allows us to make use of the past to evaluate the wisdom of particular actions and their ramifications for our future. For example, this teaching activity on timescales of climate change introduces students to the fact that climate change occurs at timescales of 1 year to 108 years and there are various drivers to explain these changes. It addresses how scientists detect these scales of climate change in the geologic record. Students, using what they learn, are then asked to evaluate the potential drivers of climate change since the Industrial Revolution.
2. Understanding geologic time is cognitively challenging.
- Students have a variety of experiences and beliefs that make geologic history unbelievable. Our everyday experience of the Earth is of an environment that is broadly perceived as unchanging, while the history of the Earth is of a planet that has changed dramatically through time. In addition, creation stories often contradict the history preserved in the geologic record. Research shows that students build on prior knowledge as they learn, even if it contradicts scientific understanding (e.g. Gobert, 2000; Taber, 2003; Chi, 2008).
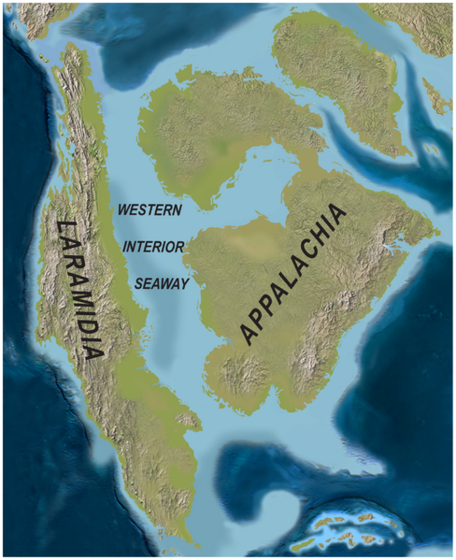
- Students do not have any personal experience, and often they come without any prior knowledge, of how the Earth has been different than its current state. They don't have a "story line" onto which they can hang events in Earth's history -- they have to build one from scratch. Given the complexity of the actual story line, it should not surprise us that students' versions fall short.
- Humans think of time primarily as a series of events, often with fuzzy boundaries (consider World War I: at what moment did it begin?). We make use of a combination of descriptive and metric information to place events in time, drawing on our knowledge of event boundaries (when events began and ended) at a heirarchy of scales. In the absence of detailed information, we use information at a coarser time scale. We also tend to underestimate the duration of events for which we have little information and to move events closer to well known, "anchoring" events in our knowledge of history. These tendencies accentuate the difficulties of students with minimal knowledge of Earth's history.
- Understanding geologic time requires thinking about processes, rates, and scales that are beyond our everyday human experiences. It involves deducing an unfamiliar history involving processes operating at a wide variety of rates over intervals of time that are far longer than we will experience as individuals. Humans and the environment we can observe directly are only a minute part of this unfamiliar history. Furthermore, the names describing the time intervals, processes and players in this story are not part of our everyday vocabulary.
- Placing events in geologic time requires working with large numbers for which we do not have an intuitive feel. When asked to place events on a timeline representing Earth history, students lack a rich set of categories into which to place those events -- they have a sense that events happened either a very long time ago or relatively recently. They tend to place events much farther back in time than appropriate, spreading them out within the history of the planet, without a sense of proportionality.
- Radiometric dating, a fundamental tool of geochronology, requires a sophisticated understanding of chemical and physical processes that students cannot observe directly.
- Synthesizing the geologic history of even a single location requires sophisticated inference that depends on an understanding of modern systems, observations of the ancient rock record in multiple places, and 4-D visualization skills.
3. While there has not been much research on best practices in teaching about time, there are many diverse and promising strategies available.

- Scaffolding student learning, an important strategy in teaching any difficult concept, can be applied to teaching temporal concepts. One key to scaffolding student understanding of geologic time is helping them to build a mental timeline for the history of the Earth. Because all of the intervals within the geologic time scale are unfamiliar, learning the names (and length, and placement) of these intervals is non-trivial. Specific scaffolding strategies:
- Work from familiar time scales to unfamiliar time scales, and have students explicitly figure out where each previous timeline fits within each new timeline. Include numerical ages at the endpoints of each timeline, and make sure that the change in scale from one timeline to another is small enough to be understood. This teaching activity on hierarchical alignment of timelines, developed by a cognitive psychologist and tested in geoscience classrooms, is designed to do just that.
- Give students a rich set of "anchors" to mark the passage of deep time, so that they can subdivide the Earth's history into many segments (rather than the two that most of them arrive with in college classrooms: recent and ancient). Help them to develop rich narratives for each of the time periods you want them to know about, so that each has its own set of characteristics.
- Explicitly help students to formulate a hierarchical structure for the subdivisions of the geologic time scale, so that they understand (for example) what ties together the periods of the Mesozoic Era or the epochs of the Tertiary.
- Explicitly address the relative proportions of the large magnitude numbers on timelines. For example, use this visualization, how many is a million?
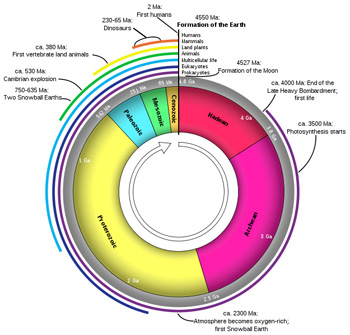
- Analogies, including timelines, are one of the most widely used techniques for teaching about the length of geologic time. Cognitive psychologists' understanding of analogic thinking provides strong guidance for the development and use of analogies.
- Spatial analogy is a common strategy for teaching about geologic time. When using a spatial analogy to represent the Earth's history, explicitly address the process of making an analogical mapping from the spatial representation to geologic time (Jee et al., 2010). Which characteristics of the spatial analogy have analogous characteristics in time, and which do not? For example, a football field has regular subdivisions; geologic time does not. Boundaries in geologic time are based on major events in Earth history. Teach about these events, and explain that they did not happen at temporally regular intervals, so that students understand that geologic time is not subdivided into regular temporal increments (unlike, for example, the length of a day).
- Use accurate analogies. For example, rather than using timelines that show the length of the Precambrian disproportionally compressed, use a timeline that helps students to visualize the Precambrian.
- A key challenge of teaching about geochronology is understanding the variety of methods we use to evaluate confidence in a geologic age. Geoscientists weave together evidence from observation of field evidence at scales from microscopic to outcrop to regional relations on a variety of scales with different chronometers measuring time on different scales. When this evidence comes together into a coherent history confidence is gained. Explicitly teaching the details of how geochronology works -- beginning with field relationships, moving to dating methods and comparing the results -- can build this understanding. For example, this teaching activity on the use of isochrons in radiometric dating has students work with real data to determine radiometric ages.
- Radiometric dating is one of the central tools of geochronology. Using manipulatives, as in this M&M radioactive decay simulation or this geochronology with beads activity, can help build an understanding of radioactive decay and the relationship between the steady rate of radioactive decay and an understanding of the age of a sample. An understanding of what make a good clock can provide an entry to this understanding and build connection to similar discussions in biology classes.
- Providing timely feedback is another general strategy that works well in supporting student learning of difficult concepts.

4. Measuring student understanding of temporal concepts is challenging, but essential. We have attempted to help others by developing
- a page about assessing temporal thinking and learning, including information about existing assessment instruments and proven strategies;
- a page of example learning goals related to teaching about time;
- a collection of assessments of temporal learning; and
- a baseline assessment, to measure students' incoming knowledge.
Developing such goals and assessments is work in progress, and we encourage our colleagues to develop and share your own assessments of temporal learning.
References:
Chi, M.T.H., 2008, Three types of conceptual change: Belief revision, mental model transformation, and categorical shift, In Vosniadou, S., editor, Handbook of research on conceptual change, Hillsdale, NJ, Erlbaum, 740 p.
Gobert, J.D., 2000, A typology of causal models for plate tectonics: Inferential power and barriers to understanding, International Journal of Science Education, v. 22, p. 937- 977.
Jee, B.D., D. Uttal, D. Gentner, C. Manduca, T. Shipley, B. Sageman, C.J. Ormand, & B. Tikoff (2010). Analogical thinking in geoscience education, Journal of Geoscience Education, v. 58, n. 1, pp. 2-13.
Taber, K.S., 2003, Mediating mental models of metals: Acknowledging the priority of the learner's prior learning, Science Education, v. 87, p. 732-758.