Initial Publication Date: October 9, 2017
×
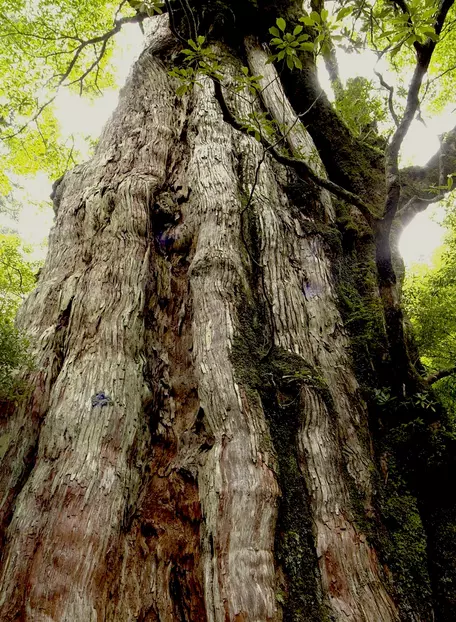
Incorporate Sustainability in your Core Teaching Content
Concepts on this page were derived from faculty discussions and presentations at multiple
InTeGrate workshops.
Bringing sustainability into your teaching offers a wide range of benefits to student learning, such as establishing relevance, bridging course content to current topics in the news, and making the course connected to other disciplines (which can also be done explicitly in an
interdisciplinary setting).
Sustainability as Part of the Trunk of your Course
Although the benefits are numerous, there are common barriers to incorporating topics of sustainability into a course, including time and content overload. Terms are short, and courses are already overflowing with core content. One method to overcome this barrier and effectively incorporate topics of sustainability is to relate them to the core content, and not as course "add-on's" - part of the "trunk" of a course, not out on a twig. The Curriculum for the Bioregion project identifies this "big ideas" method as one of a few promising pedagogies for teaching sustainability.
How to Identify Target Opportunities
Incorporating sustainability into the core content of your curriculum begins with identifying:
- disciplinary core concepts that you already teach that might lend themselves to sustainability connections and contexts
- sustainability concepts that could be usefully integrated into your course
×
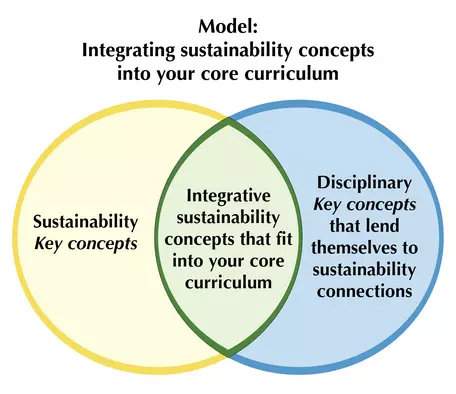
Click to enlarge image.
×
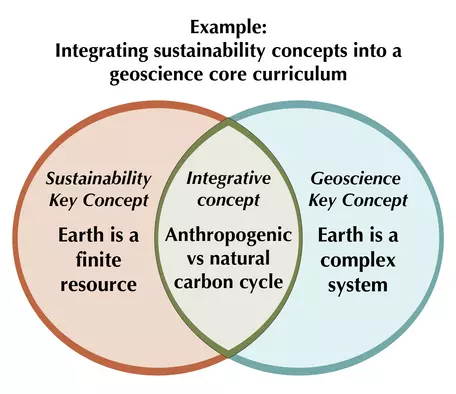
Click to view larger. Anthropogenic versus the natural carbon cycle overlaps with Geoscience, but could also overlap with core concepts in Biology (cycling of matter), Chemistry (Mass-balance), Engineering (life-cycle assessment), Geography (importance of place and scale), and so on.
Example Core Disciplinary Concepts that Might Lend Themselves to Sustainability Connections
Participants from several of the the 2012-2013 InTeGrate workshops worked with others in their discipline to generate a list of key concepts that are essential for students to learn and also allow opportunities to bring sustainability into the curricula. What are the essential concepts that students in various disciplines should learn? How do concepts from different subject areas overlap? Where are the opportunities for teaching about sustainability across all disciplines?
- Systems thinking and the relationship between systems
- Energy flow
- Cycles, budgets and feedbacks
- Natural resources: where they come from, implications for use, limitations of resources
- Human interactions with Earth's systems
- Cultural influences, values, ethics, political systems
- Resilience
- Risk
- Decision-making
- Interdisciplinary and the importance of collaboration
- The importance of place, especially one's local place
- Process of knowing, whether from the scientific, cultural or historical perspective.
- Diversity of life - relevance to ecosystem resilience globally. It is essential for the resilience of the ecosphere and also for agricultural resilience in an era of monocropping.
- Unity of life - Precautionary principle: small changes can have unintended, widespread consequences. This has been an issue that has been raised with genetically modified organisms.
- Cycling of matter - sustainability issue for nitrogen and phosphorous in agricultural systems. Human, matter, and energy flow interact. The impact of humans on these natural processes is at the heart of sustainability challenges.
- Energy flow including photosynthesis, respiration, trophodynamics, energy subsidy (hawk soaring) - all life depends on solar energy which moves between trophic levels after photosynthesis with a 10% efficiency. This has implications for feeding the world (importance of eating low on the food chain).
- Evolution - life is constantly evolving and humans are substantially affecting the course of evolution through anthropocentric effects, including artificial selection.
- Natural and artificial selection (e.g. all of agriculture)
- Ecosystems have abiotic and biotic components (community plus physical environment)
- Growth and development for individuals and populations
See the work of the biology community in Vision and Change in Undergraduate Biology Education for core concepts (http://visionandchange.org/). Also consider the core concepts in the new AP Biology curriculum and in the new Framework for K-12 Science Education that is driving the Next Generation Science Standards (http://www.nap.edu/catalog.php?record_id=13165 and http://www.achieve.org/next-generation-science-standards).
- Atomic structure - isotope abundance: atmospheric composition, fossil fuel tracing, geological aging/historical climates
- States of matter - energy: dependence on solid, liquid and gas forms of fossil fuels
- Periodicity - abundance of elements, mineral sourcing (cell phones, lithium batteries, etc.), geo-political stability of sources
- Mole concept, stoichiometry, chemical equations, mass balance → carbon emissions, anthropogenic/natural carbon cycle, carbon sequestration (limiting reagent: limiting nutrient for phytoplankton blooms in ocean and quantifying carbon sequestration/ iron seeding)
- Molecular structure (VSEPR, MO, bonding) - Greenhouse gases and IR absorption, bioaccumulation (solubility)
- Quantum concepts - light absorption, photovoltaic cells, photo synth, analytical instrumentation - quantifying pollutants in the environment
- Gas Laws - carbon sequestration, hydrogen fuel production/storage/combustion, combustion engines/Carnot cycle
- Equilibrium - nutrient cycling (biogeochemistry), Born-Haber reaction and agriculture, smoke stack scrubbers, detection of analyses in the environment - design of sensors
- Acid/base - natural buffers, acid rain, ocean acidification, acid mine drainage, metabolism of bioaccumulative compounds
- Solution chemistry/Solubility - water pollutants: sources, fates, of pollutants, quantifying concentration, carbon dioxide uptake by the oceans
- Thermochemistry/chemical kinetics - Energy, fuels, combustion, conversion efficiency of engines/devices, excited state decay - solar PV, catalysis and "green chemistry"
- Redox chemistry, electrochemical potential - western dependence on battery power/electrical energy , hydrogen production from water, fuel cells
- Nuclear chemistry - ore, refining fuels, storage, nuclear power and wastes (decay/lifetime), leeching of radioisotopes
The list above was organized as a traditional General Chemistry, two semester course. The list highlights where and how environmental and sustainability issues can be used to achieve teaching objectives.
- Understand Earth through repeatable observations
- show how to think and act like a geologist
- careful observation of natural systems helps us understand processes and interactions within those systems
- Earth is a dynamic, constantly changing planet
- Earth's structure/plate tectonics and its relationship to volcanoes, earthquakes, mountain building, etc.
- atmospheric evolution
- Earth's history
- coastal processes (constantly changing as result of interactions of climate/weather, tides, human interactions, biosphere, etc.)
- Temporal and spatial scale
- geologic time
- Earth processes on human time scales compared to geologic timescales
- applicable to cycles (residence times)
- examples: resource development time
- SIZE: smallest to largest (bacteria vs. whales, ocean bathymetry vs. continental topography,reservoir sizes
- Systems: reservoirs, fluxes, sinks, sources, transport mechanisms, and residence times
- also includes feedbacks
- specifics: water storage and movement, currents, pollution, heat, sediment, nutrients, ions, water
- applicable to cycles
- open vs. closed systems
- Interaction among Earth's systems (biological, hydrologic, lithospheric, atmospheric, interior of the Earth, etc.)
- interconnections and feedbacks among systems - positive/negative
- carrying capacities
- specifics: climate controls, ecology (creation of an environment), pollution
- Human interaction with planet - Resources, Hazards, and Management
- creation of resources and hazards and how humans use/interact
- all life depends on resources provided by the planet
- specifically in regard to oceans' resources: fishing/farming/sand use, etc.
- description of the anthroposphere and its interactions (politics, culture, society, etc.)
- Scientific process/uncertainty
- Process understanding
- resource distribution and allocation
- Tectonics
- Nature of science
- Relevance (human aspect of geoscience)
- Non-unique solutions
- Interconnectedness, connectedness: emphasis on relationships (people and environment, different places, different systems)
- Importance of place and scale: sustainability will look different in different places (physical and social environments vary); sustainability will also "look different" and need to have different emphases based on scale (global vs. regional vs. local)
- Landscape (a place concept) and landscape dynamics. Reading it, connecting to it. (Phenology is one approach; access to "nature" or to broader landscapes matter.)
- The meaning and interpretations of sustainability.
- Purpose(s) of and approach(es) to sustainability science.
- "Sustainability" is normative: it will involve choices as to what to sustain (and where).
- There are different strategies depending on place (and scale) and different normative choices and values.
- Systems = a crucial concept. To understand systems and system change, we also need to understand driving forces.
- Global change: climate, population, biota, economic interdependencies.
- Resilience.
- Themes of human-environment relations (H-E studies): human impacts on the environment, environmental perceptions, and hazards (the main theme related to environmental impacts on humans).
- Mapping as a practice to help with understanding relationships; data analysis (including spatial data)
- 5 themes of geography (NCGE/AAG; 1984):
- Human-environment interactions
- Scarcity and the coordination of scarce resources with human wants.
- Production, costs, and technology. E.g. direct vs. indirect costs and internal vs. external costs.
- Social institutions (rules and norms) shape incentives.
- Incentives shape the behaviors of consumers, firms, and government.
- Competitive environments (e.g. firms, consumers, government) impact market outcomes and resource use.
- Systems thinking: everything is connected, in context, and interrelated
- Interdisciplinary: no sustainability problem comes from a single discipline, and no solution will either
- Scale: temporal, spatial, and physical scale are critical to evaluating problems and solutions
- Critical thinking in context: knowing which questions to ask
- Application of these concepts in geoscience: water, energy, mining, climate, restoration/remediation
- Cycles and budgets (including pools and fluxes, e.g., biogeochemical cycles)
- Process of science, including issues in subsampling, extrapolation, uncertainty, predictability, stochasticity
- Dynamics and the influence of history: legacies, alternate stable states, hysteresis, equilibrium
- Natural resources and their limitation
- The role of social, political, economic and cultural forces in determining resource extraction, allocation, consumption, transport
- Basic principles: (1) Principles of ecosystem sustainability; (2) Nutrient cycling in human & natural systems; (3) Energy flow in natural systems; (4) Population growth models
- What is sustainability?
- This is a foundational concept with multiple definitions. The concept has different meanings in economics and ecology contexts. It's important for students to have familiarity with the multiple meanings of this concept. For our purposes however, the meaning of sustainability as it is applies to ecosystems is most applicable. The list enumerated below outlines key concepts of ecosystem sustainability that form the basis of sustainability science.
- The Human Dimension: Civic engagement & environmental justice. One aspect of sustainability science that differentiates it from environmental science is that it incorporates human social, political, and economic systems. Although deep inquiry into these topics would be beyond the scope of an introductory course, students should have some opportunity to explore the human dimension of sustainability science through opportunities to participate in civic engagement, such as through community-based learning opportunities or through exploring topics relevant to environmental justice.
- Connection to Science: Process of scientific inquiry; Science communication to varied audiences; Concept of uncertainty in science - this is relevant to sustainability and important to address in all introductory science courses.
- Systems thinking
- Whole-systems based education - not only discipline-based education
- Teaching complexity and getting students comfortable with complexity
- Understanding that the biosphere sustains the economy (i.e. dysfunctional biosphere = dysfunctional economy)
- Integral to life cycle analysis; ecosystem services; ecosystem (natural) capital, biogeochemical cycles.
- Teaching collaboration as stepping outside our discipline into whole systems conversations, rather than pulling other disciplines into a discipline-framed conversation
- Sustainability as a way of thinking
- Beyond peer evaluation
- Balance peer evaluation (i.e. "people that think like you") with beyond-peer evaluation (i.e. "people who think beyond the discipline's worldview")
- Optimization of everything rather than the maximization of one thing
- Metacognition
- Two levels of change (incremental change disciplines as interim strategy; radical change in education as path to sustainability)
- e.g. the "unsiloed university"
- Incremental change within the current discipline-based university while we take down the "silos"
- Focus on local
- Physical location - working in the local area
- There should be hands-on components (helps with empowerment)
- Mental - building student confidence that they can affect change
- Have students assess their own activity against the standard (e.g. carbon, ecological, and water footprints)
- Teaching to hope
- Major emphasis on solutions
- Envisioning a sustainable future
- The nature of knowing (knowledge systems)
- Include science as one of those - but also traditional, formal/informal
- Appreciating multiple worldviews and knowledge system
- Resource use vs. waste
- Interdisciplinary
- Social and ecological systems
- Information access
- Values in use
- Values vs. information
- Livelihood
- Risk
- Resilience
- Life cycle assessment
- Metrics
- Back of the envelope problem solving
- Working across scales
- Information access
- Linear vs. non linear change
Big Ideas, Learning Outcomes, Skills, and References Relevant to a Sustainability Course or Curriculum by Robert J. Turner, Assistant Professor of Environmental Science, University of Washington, Bothell
Wiek, A, Withycombe, L., Redman, C.L.,
Key competencies in sustainability: a reference framework for academic program development , Sustainability Science, July 2011, Volume 6, Issue 2, pp 203-218
- Supply and demand - balance, optimization
- Risk and reliability - design criteria (e.g., sizing, resistance, capacity), disaster/hazards planning and mitigation
- Systems thinking - cradle-to-grave, cradle-to-cradle, life-cycle assessment
- Quantitative skills - maps, graphs, GIS
- Ethics - ethical responsibility of engineers, public safety
- Multi-criteria decision making (Triple Bottom Line, Five Pillars)
- Construction materials - embodied energy, material flow analysis
- Materials - green manufacturing, product substitution
- Natural resources - water, land/soil
- Environmental quality - air, water, soil - fate and transport, impact assessment
- Transportation - logistics - hazards, economics, equity
- Restoration - water/wastewater treatment, air pollution control, stream channel rehabilitation
- Infrastructure - life cycle, planning, design, construction, operation and maintenance, and decommissioning\
- Urban and community planning in general as well as anticipating and in response to natural hazards
- Coastal geology/engineering: climate impacts, sea-level change, coastal aquifers
- Improving public science and engineering literacy
- Energy - including energy policy, priorities, sustainable development, usage, and managing the transition from carbon-based energy to the next generation
- Ensuring supply of potable water
- Ensuring safe reliable food supply; soil conservation, urbanization
- Adaptation and mitigation strategies for the effects of climate change
- There are other legitimate ways of knowing outside of the scientific paradigm, that includes our bodies and our histories.
- Introducing humanistic considerations into the conversation:values, power structures, ideology, etc.
- Questions about who, what, when, and why are vital in discussing sustainability.
- Interdisciplinary nature of oceans in general - biological, physical, and chemical components and their interactions
- Impact of shipping on the world economy - (e.g. a class debate on cost benefit of shipping and the balance between economic need and environmental consequences)
- Coastal management, sand resources, and control structures, including the impact of human constructs on the flow of sediment; the complexity of the questions around coastal "management": e.g., should we pay for beach replenishment - what does this cost, what economic development is encouraged by replenishment, will construction of groins, jetties, etc and of replenishment, be cost-effective? What is the impact on the down-sediment stream?
- Aquaculture, including using aquaculture to investigate cultural differences, history of the development of aquaculture, comparison between agriculture and aquaculture, environmental issues related to aquaculture, costs and benefits of aquaculture
- Interactions between humans and the ocean
- Mineral and energy resources
- Fisheries and aquaculture
- Marine pollution
- Describing ocean chemistry and processes of nutrient cycling
- Relate scales and rates of ocean and ocean processes
- Ocean and climate change - how physical and chemical factors in the ocean affect the climate in the past, present and future
- Role of policy, carbon trading
Example Integrative Sustainability Concepts
There are a large spectrum of ways one can approach integrating sustainability into a course. Although this task may be daunting, it's been done before and you can benefit from example materials from your colleagues.
InTeGrate Developed Modules
Activities
Courses
Interdisciplinary themes
Click to view
Ethanol & Sustainability Teaching: Integrating Business, Public Policy, and Science (PowerPoint 2007 (.pptx) 3MB Jul25 12)
Rick Oches, Bentley University