For the Instructor
These student materials complement the Renewable Energy and Environmental Sustainability Instructor Materials. If you would like your students to have access to the student materials, we suggest you either point them at the Student Version which omits the framing pages with information designed for faculty (and this box). Or you can download these pages in several formats that you can include in your course website or local Learning Managment System. Learn more about using, modifying, and sharing InTeGrate teaching materials.Student Reading: Passive Designs
Passive designs for optimizing with nature: smart building designs to conserve energy
Learning goals: Students will be able to:
- Recognize and explain the four basic principles of passive design.
- Explain the different types of energy and the application of basic thermodynamic principles as applied to the design of buildings.
- Distinguish between active and passive design at different building scales.
- Identify the site-specific roles of orientation, insulation, ventilation, shading, and window type and placement in optimizing passive design.
Learning assessment: Students interpret a graph showing heating energy costs versus degree days. Students compare the thermal properties of different building materials for energy conservation. Students complete a computer-based visual exercise to apply knowledge of passive building design.
Green Buildings and Optimizing with Nature
In an effort to reuse throwaways and recyclables, some people have taken to building homes. Thus, one can find examples of houses made of glass bottles, aluminum cans, tires, and other materials. One can certainly argue that use of materials that might otherwise end up in a landfill is wise use and a smart way to design a building. Some of the materials, in addition to being cheap and easily obtained, form barriers to heat flow and are energy-efficient.
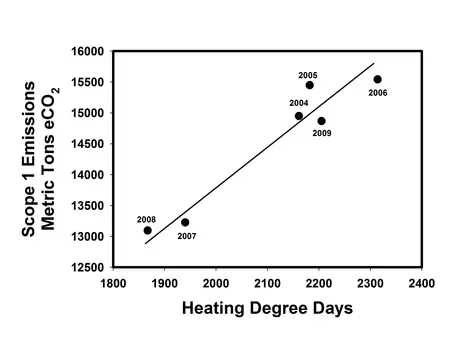
The heating degree days are plotted against the "scope 1 emissions"—greenhouse gas (GHG) emissions of equivalent carbon dioxide (eCO2) generated by the burning of fossil fuels on campus from 2004–2009.
Whether considering an entire college, a home, a business, or any individual building, outside temperatures tend to vary, and those temperatures may be above or below some optimum inside temperature (65-70° F, or 18-21° C), depending on day, season, or geographic location. The costs to adjust inside temperatures up or down with heating or cooling can be substantial.
What to do? A number of options are available to heat and cool buildings, but one of the simplest methods for energy conservation is passive and has to do with initial building design within the context of local landscape (geospatial) and regional climatic/meteorologic features. The idea is to design with nature in mind, to exploit the unique heating/cooling benefits of individual sites. Reptiles, amphibians, insects, and other ectotherms have figured out ways to exploit their environment for external sources for heating and cooling, so why can't we? Here, we consider some aspects of climate responsive design.
Passive design strategies are based on four basic principles, notably 1) climate analysis and comfort; 2) passive heating; 3) passive cooling, and; 4) daylighting. Clearly, many of the passive design strategies involving heating, cooling, and daylighting are dependent upon climate, and thus climate analysis is of foremost importance to the development and application of passive design technologies.
Various elements are associated with effective, passive designs for energy conservation. For this module, we focus on variation in thermal energy from solar, and so consider the following aspects with respect to passive solar design.
1) Siting (location, location, location): Earth rotates on its axis, so the amount of incoming solar radiation varies on a day-night basis: in the most general way, heating occurs by day and cooling by night. Further, Earth is a sphere, and the amounts of incoming solar radiation absorbed at any latitude will vary with respect the curvature of Earth. The incoming angle of radiation is almost directly perpendicular to Earth's surface near the equator, but angled much less directly nearer the poles. More light is absorbed and more heat is generated to the south, near the equator; less light is absorbed and more is reflected near the poles:
For a solar system in which Earth spun on an axis perpendicular to its rotational orbit around the sun, then this differential heating of Earth would be the big story day in and day out. But wait, there is more! Earth is tilted on its axis some 23.5 degrees, so that the angle of exposure to the sun's rays varies seasonally as Earth completes its annual cycle around the sun. During summer in the United States, for example, the northern hemisphere is tilted toward the sun, the incoming angle of solar radiation is more direct, and more solar radiation is absorbed. During winter in the United States, the northern hemisphere is tilted away from the sun, and so there is less heating potential. The number of daylight hours (and solar heating potential) increases and decreases, respectively, during summer and winter.
OK, so what are the ramifications of these celestial factoids, and how can they be applied to passive site design for energy conservation? One has to be aware of the relative amount of solar radiation available for heating and how to use it. In the northern hemisphere, solar radiation for latitudes above the Tropic of Cancer (at 23.5 degrees north latitude) is more intense to the south, so orienting a building or energy-capture devices to the south is an important first step. This is especially important during the cold winter months so that the building can take advantage of a southern exposure. During the summer, of course, a southern exposure can lead to unwanted heat generation, so methods for cooling—not heating—are required during summer. Obviously, solar "availability" is not the only aspect of climate (the #1 passive design principle), as prevailing weather conditions at similar latitudes can vary greatly owing to factors in addition to solar (topography, adjacency to open water, altitude, etc.).
2) Windows: Beyond location and orientation, the building should take advantage of its solar exposure. Windows let the sunshine in, and the glass traps the heat energy inside the building, so having windows exposed to sunlight on the south side of the building is a good thing. South-facing windows typically comprise less than 10% of a building's total floor space, but may provide heat to the entire building. This type of thermal heating from solar is called direct gain, because the incoming light warms the interior space—a valuable source of heating of window facades on buildings. Window glazing can improve retention of thermal energy from solar during winter and minimize retention during summer. Ongoing advances in window glazing with double- and triple-pained glass continue to improve the design of windows for climate responsiveness.
3) Construction Materials: As an added potential benefit, construction materials of the inside and outside space that have high heat capacity can warm during the day and then re-radiate that heat at night after the sun goes down. Increasing the thermal mass or solar mass of the building (i.e., increasing the ability to store and re-radiate that thermal energy derived from solar) is another conservation strategy for reducing the need for burning fossil fuels for heating. Concrete and brick have the capacity to absorb heat and re-radiate slowly back into the interior space. Wood, particle board, and drywall typically are thinner, less-dense materials and thus have lower thermal mass. Use of naturally occurring construction materials is an added benefit, relative to using materials derived from petroleum byproducts or other chemically-engineered sources.
With the objective to reduce heat loss in the winter and heat gain in the summer, the building materials used for the roof, walls (inside and out), floors, and windows are critical—materials that can provide good insulation. To that end, thermal insulation and envelope tightness are essential passive-energy conservation measures that maximize passive heating. Building materials with high R-values (a measurement of resistance to heat flow—the higher the R-value, the better the insulation) should be utilized for roofs and walls.
4) Other Modifications: Similarly, the design of interior space and the exterior space can contribute to optimizing with nature. These designs might include:
Trombe Walls: Trombe walls are south-facing house walls made of materials that retain thermal mass when heated during the day. The wall then radiates that heat during night, thereby stabilizing internal house temperatures. You have done the experiment with the canning jars—which building materials make for good heat retention? The wall typically is faced externally with glass, with a closed air space between the glass and wall. During the day, incoming solar radiation in the UV range heats the wall; as near-infrared heat is radiated off the wall, it is effectively blocked from radiating through the glass (the greenhouse effect). Instead, the heat radiates into the house. Obviously, a dark-colored wall will absorb more solar radiation than a light-colored wall that reflects.
A modification of a Trombe wall is a solar air heating system, for which the warm air in-between the wall and glass is circulated passively into the house. Since warm air rises, the wall is open to the interior of the house, allowing warm air to move by convection into the house. The air in front of the wall is replaced by cooler air. From where would you draw cool air? Solar air heating systems use the heated air right away via convection (versus Trombe walls that radiate heat stored in the thermal mass of the wall).
During summer, the Trombe wall would be a liability, not an asset! Shading of the wall via a roof overhang can block the solar rays from high in the sky during summer days. Likewise, shading of windows with awnings or shutters can keep a building from overheating during summer.
Solar Chimney: A special type of solar air system is the solar chimney. Solar chimneys, as the name implies, typically are located atop buildings, where solar energy heats the chimney and enclosed air. As that air vents to the outside above the level of the house, cool air is drawn into the house from lower levels, thereby providing ventilation inside the house.
×Landscaping: Building orientation is critical to passive solar heating and cooling, as already mentioned. Consider the different sides of the building:
- East-facing walls receive intense morning sun;
- South-facing walls are exposed to the sun throughout the day;
- West-facing walls receive intense afternoon sun;
- North-facing walls are shaded from the sun throughout the day.
Although the south-facing walls are exposed the longest to the sun, they do not necessarily get the hottest. Which walls do?
During winter, the south-facing walls receive the most solar radiation and thus are most important to passive heating from solar radiation. Daylighting, of course, is an essential design principle, as daylight connects people with the outdoors, provides views, and minimizes dependence on artificial lighting. But what about during summer—how does one deal with all the heating potential if the last thing you want is more heat? Use shading—a classic passive cooling principle! For example, one can include shading in the architectural design of the building. In addition to natural ventilation and using ventilation techniques like solar chimneys, landscaping can be used to decrease solar radiation through shading, thereby providing more passive cooling. The planting of trees strategically placed on the land surrounding the building can help shade the building. Which types of trees would you use around a building (deciduous or evergreen), and where would you plant them? Trees typically are selected based on their growth form, canopy density, and height. Finally, native trees are best since those species are the ones that are adapted to the climate where the building occurs.
Floor Plans: In addition to window and wall orientation to exploit the seasonality of the sun, floor plans can be designed/modified to improve access to heat via conduction (ex.: the direct gain of a sun-warmed floor in a sunroom heats your feet), convection (ex.: warmed air from a solar space is redistributed throughout the house), and radiation (ex.: the indirect gain of a heated Trombe wall re-radiates heat into an interior room). Obviously some areas of the house will be sites that collect solar heat and others will not, so keeping an open floor plan can allow for collected heat to circulate more freely throughout the building via natural convection. If the building is longer along the east-west axis, then more rooms potentially can face south to the sun; typically, these rooms should include high-activity rooms like living rooms where people congregate and will benefit from incoming solar heat. Rooms that are not used as frequently should be placed along the north side of the building, thereby acting as buffers from the cold (i.e., closets and storage rooms). Hot water heaters are connected by pipes to where water is used (bathrooms, kitchen, and laundry rooms), so clustering these areas near the water heater decreases heat loss along lengthy water lines.
Together with additional solar-driven options like photovoltaic arrays and solar water heaters, a passive solar building can be quite active in its well-planned use of daily and seasonal variation in incoming solar radiation.
For more information:
- Passive Solar Design Primer from the New Mexico Solar Energy Association (p. 71)
- Passive Solar Home Design article from Energy.gov
- DeKay, M. and G.Z. Brown. 2013. Sun, Wind and Light: Architectural Design Strategies. John Wiley and Sons.
- Givoni, B. 1992. "Comfort, climate analysis and building design guidelines." Energy and Buildings 18:11–23