How big was that quake?
Quantitative concepts: probablility, recurrence intervals, logarithms
by Dr. Eric M. Baer, Geology Program, Highline Community College
Essential Concepts
Generally there are several main concepts that our students struggle with when thinking about earthquake magnitude and size:
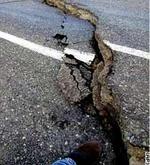
- the difference between earthquake magnitude, damage (intensity), and shaking,
- quantitatively measuring earthquake size including magnitudes, moment, and energy released,
- measuring earthquake damage, including intensity and the factors that determine intensity,
- how to measure earthquake shaking
The difference between earthquake magnitude, intensity, and shaking
Ask many seismologists what the most critical and common misunderstanding about earthquakes is and they will answer "the difference between magnitude and intensity." Earthquake intensity and magnitude measure different things and are often misunderstood, and it is shaking that links them.
Earthquake intensity is a measurement of damage. Earthquake magnitude is a measurement of the "size" of the quake - typically related to the amount of energy released. There is one magnitude for an individual quake, but the intensity varies significantly. Earthquake shaking is typically measured as an acceleration; higher magnitude earthquakes cause more violent shaking, which in turn typically cause higher intensity.
People often think that magnitude of the earthquake is the only factor that determines damage. Typically this will be shown by someone asking if a particular building will withstand a "magnitude 8" earthquake - and ignoring the distance, duration, hazards other than shaking, and other factors.How big was that quake? magnitudes, moments, and energy
When one asks "how big was that earthquake?" the answer is qualitative because it depends on what is meant by "big." There are several ways that the size of the earthquake can be measured quantitatively. The size of earthquakes is typically given as a magnitude.
Earthquake magnitude: MB, ML, MS, Mw - and what is the Richter Magnitude anyways?
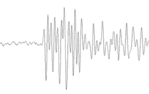
There are at least 4 different measurements of magnitude in use today. This causes quite a bit of confusion. The most popular and well known is the Richter magnitude (ML), also known as the local magnitude. It is also the worst of the four. The body-wave (MB) and surface-wave magnitudes (MS) are offshoots of the Richter magnitude using those particular waves only. Finally, the fourth and perhaps least known is the best estimate of the size of the earthquake, the moment magnitude (Mw)
The Richter Magnitude
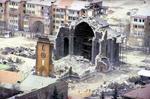
Charles Richter developed the Richter magnitude in 1935. It is defined as the logarithm of the amplitude (measured in thousandths of millimeters or microns) of the largest seismic wave measured 100 km from the epicenter on a particular brand of seismometer. The definition is an excellent place to start dissecting the problems with the Richter magnitude.
The first part of the definition creates the most confusion. The Richter scale is logarithmic, meaning that for each increase in the magnitude there is a 10-fold increase in the shaking. Few people understand this and it leads to substantial problems.
The logarithmic nature of the Richter magnitude isn't the only source of this scale:
- The Richter magnitude measures the largest seismic wave no matter what type it is p, s, or surface (For more information on seismic wave types see the seismic waves page). Duration does not mater.
- ML also is defined for seismograph 100 km from the epicenter , which is unlikely. Error-prone corrections must be made to estimate what the shaking would have been 100 km from the epicenter.
- The model of seismograph that Richter used is no longer in service and so other seismographs have to be calibrated to Richter's standard; this model was particularly sensitive to waves of one frequency, so earthquake magnitude was often determined by this single frequency waves.
Moment magnitude
The moment magnitude (Mw) scale is the most common magnitude scale in use among seismologists today, and seems to more reliably indicate the size of the earthquake than other. The moment magnitude is based on the seismic moment, which is determined by multiplying the amount of slip on the fault, the size of the fault plane, and the strength of the rock. This gives a result that is closer to measuring the amount of energy released by the earthquake. The moment magnitude is also logarithmic, however, and thus has some of the same drawbacks as the Richter magnitude. It is also somewhat harder to measure.
The difference between the magnitude scales based on seismic wave amplitude and the moment magnitude is best illustrated by looking at two huge subduction zone earthquakes, the 1960 Chilean quake and the 1964 Alaska quake. On the Richter scale, the Alaska quake was "bigger" having a MS of 8.6 compared to the MS of the Chilean quake of 8.5. However, in measuring the seismic moment, the Chilean quake was larger, giving Mw of 9.5 versus the Mw of 9.2 for the Alaskan earthquake. The reason is that the Chilean earthquake released more energy, but in the Alaskan earthquake the energy was transmitted more efficiently through the Earth, causing more shaking at 100 km distance.The seismic moment
While most introductory students have not encountered moments before, I find that they can understand the concept of a seismic moment. It is only the name that stymies them! The seismic moment (MO) isMO = µAd
where µ is the shear modulus of the rocks along the fault, A is the area of the rupture along the fault, and d is the average displacement along the fault.
Seismic energy
Whereas seismic moments are more precise, students believe they understand the measurement of seismic energy better - the amount of energy released by a quake. The measurement of energy does allow earthquake size to be related to some events that are more familiar to some students. For example, the great Sumatran earthquake released 5x1025ergs, which is the energy released by 1200 megatons of TNT. The largest US nuclear weapon had a yield of 25 megatons TNT.
None of the four magnitude scales, the seismic moment or even the amount of energy released measure what most people want to know, and that is how shaking occurred, and how much damage was done.
Earthquake intensity - the real measure of the "badness" of the earthquake
Earthquake damage is measured on an intensity scale, the most common being the Modified Mercalli Intensity (MMI) scale.
The Modified Mercalli Intensity scale is based on human observations of damage and effects of earthquakes, not any measurement by a machine. In fact, on their web site, http://earthquake.usgs.gov/earthquakes/dyfi/ 'the U.S.G.S. currently requests damage reports' from anyone who has felt an earthquake. This information is gathered to produce an intensity map quickly and from many sources.
Each earthquake event has a wide range of intensities, even though each event has a single magnitude. Intensity is determined by a number of factors including:
- the amount, duration, and frequency of seismic shaking
- building construction
- nature of non-shaking hazards (such as liquefaction, tsunami, and landsliding).
The MMI scale has some obvious advantages but is not without its problems. It is somewhat subjective; some areas have characteristics of two or more intensities; an intensity map may take a long period to develop; and finally, scale is not always appropriate to all areas of the world with different building practices. Despite all of these detractions, a good intensity map is probably the best way to answer the question "how bad was this quake?"
Intensity is typically shown on an isoseismal map - a contour map of the intensity. I find construction of these maps as an in-class activity (see below)is an excellent reinforcement of the concepts of contour maps that I emphasize in other parts of the course, including topographic maps.
Measuring shaking - the primary determinant of damage and intensity
Shaking is one of the primary determinants of damage, and is something that most students can relate to but is rarely dealt with quantitatively. This is a shame, since it is an excellent opportunity to tie the clear consequences of an earthquake to the fundamental equations of physics.
Shaking in an earthquake is measured in a variety of ways, but the most common single measurement is the maximum shaking, typically measured as a peak ground acceleration (PGA). Other units that are important may include peak ground velocity, duration of shaking, and the spectraldistribution of seismic shaking. Acceleration is often the primary consideration in looking at shaking because it determines how much force an earthquake impacts on a building, and thus if a building will stand. Many students may be familiar with the equation
F = m a
Units of shaking: g isn't grams!
Accelerations are typically measured in units of g, where g is the acceleration due to gravity on the surface of the Earth. An acceleration of 1.0 g would alternatively throw you up in the air and slam you to the ground with twice the force of gravity alone. This rarely occurs in earthquakes, and would cause catastrophic damage if it occurred for any length of time. Typical accelerations in earthquakes are between 0.05 and 1 g. 0.005g would be barely felt (and not felt by many), shaking over 0.05 g is unpleasant, and few buildings would survive an acceleration of more than 0.5 g. Since shaking is almost always less than 1 g, it is also reported as a percent of g. Thus shaking of 0.1 g would often be labeled 10% g.
Since shaking is one of the primary causes of damage, there is a clear trend of higher accelerations causing greater damage and therefore greater intensities. As a result there is a correlation between acceleration and Mercalli Intensity.
While the peak acceleration is important, and is indeed the crucial number that many, if not most, engineers design buildings for, it is not the only control on damage. The duration of shaking is also important because the materials that buildings are made of, especially steel, can become fatigued. So a structure may withstand a force of 0.2g for a short while, the bending that the movement causes weakens the edifice until it might fail. This can be easily seen by bending a paper clip many times. Never is any more force being applied to the clip, but each time it is bent, it is easier to bend it until it breaks. This is a particular problem in large buildings that are designed to sway in earthquakes. Swaying initially is generally good as it dissipates the seismic forces, allowing the building to stand, however the swaying can lead to catastrophic failure due to fatigue.
Teaching Examples
- Earthquake Shaking and Damage This is a student homework exercise designed to familiarize students with earthquake shaking, acceleration, intensity and hazards, including the quantitative measurement of these properties. Students examine quantitative measurements of earthquake hazard from the U.S.G.S. then use these numbers to determine the damage to their homes (or another location) that could be inflicted by a quake with a 500-year recurrence interval (10% probability of exceedence in 50 years). By using students' own homes, they see the impacts of shaking, hazard, and intensity in a more personal, connected way.
- Earthquake Shaking—Building Contest and Shake Table Testing Activity This activity has students build buildings and test to see how resistant to shaking they are. Using the recommended accelerometers, it can give a student an excellent idea of why shaking matters.
- The Richter Scale Activity This interactive macromedia page allows students to use a nomogram to determine the ML for 4 different quakes.
Resources
There are too many excellent resources on the subject of the size of an earthquake to list all here. However, some good starts:- Earthquakes (5th Ed.) The best single reference on this topic written for the non-geophysicist.
- http://earthquake.usgs.gov/learn/index.php 'Learning and Information from the U.S.G.S. Earthquake Hazards Program' This entry into the educational part of the U.S. Geologic survey includes links to earthquake facts, a glossary, and resources for students and teachers.
- Several U.S.G.S. fact sheets discuss these topics:
- "ShakeMaps"-Instant Maps of Earthquake Shaking
- Earthquake Shaking-Finding the "Hot Spots"
- Did You Feel It? Community-Made Earthquake Shaking Maps
- The Severity of an Earthquake Describes magnitude and Mercalli intensity scales.
- Investigating Earthquakes through Regional Seismicity This module has a great deal of information about earthquakes including many very nice activities. Module 3 is particularly relevant to these topics.