Introduction to Nanoscience: Some Basics
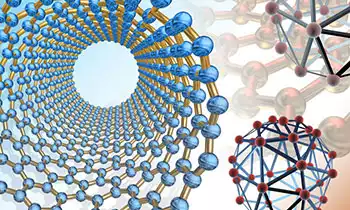
See the online presentations on:
- Nanotechnology 101 Lecture Series. A good place to start is the presentation on A Gentle Introduction to Nanotechnology and Nanoscience which "...examines the fundamental issues underlying the excitement involved in nanoscale research - what, why and how. Specific topics include assembly, properties, applications, and societal issues" (posted at nanoHUB).
- Another great introductory resource is Nanotechnology 101 from Nano.gov includes: What it is and how it works, What's so special about the nanoscale?, National Nanotechnology Initiative (NNI) Accomplishments Archive, Nanotechnology Timeline, and a Glossary.
- For resources at the graduate level, go to Nanotechnology 501 Lecture Series
Nanoscale--How Big?
A nanometer is one-billionth of a meter. The National Nanotechnology Initiative provides some excellent comparisons of materials on different scales at Size of Nanoscale. The following are some graphics that demonstrate the relative size of numerous types of materials ranging from the nano- to the macro-scale. Nanomaterials have at least two dimensions that are between 1 and 100 nanometers in size. On this scale, interatomic (coulombic) forces become large, and must be considered when undertaking studies to characterize, experiment, and model the behaviors of nanomaterials.
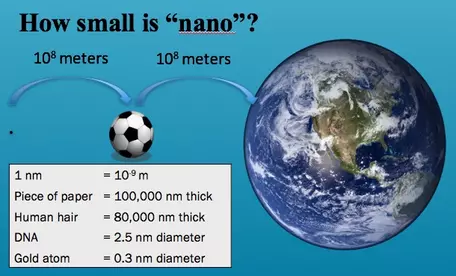
Types of Nanoparticles
Nanomaterials (NMs) are functional materials consisting of particulates with at least one dimension below 100 nanometers (nm) (Grimsdale, A. C., and Müllen, K., 2005, The chemistry of organic nanomaterials: Angewandte Chemie International Edition, v. 44, no. 35, p. 5592-5629).
Hochella et al. (2019) Natural, incidental, and engineered nanomaterials and their impacts on the Earth system, Science, March 29, 2019, provide the following definitions:
- Natural nanomaterials - A nanomaterial made by nature through (bio)geochemical or mechanical processes, without direct or indirect connection to a human activity or anthropogenic process.
- Incidental nanomaterials -A nanomaterial unintentionally produced as a result of any form of direct or indirect human influence or anthropogenic process.
- Engineered nanomaterials -A nanomaterial conceived, designed, and intentionally produced by humans. (Nanoparticles are being produced in any number of processes, and they are at large in the environment. We just haven't had the means to look for them—but here they are, and they are doing work!)
- Anthropogenic nanomaterials -Both incidental and engineered nanomaterials.
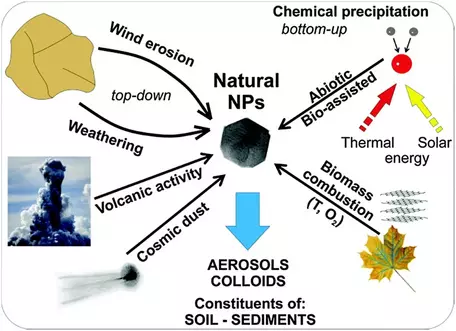
- Nanominerals are defined as minerals that only exist in the size range of approximately one to a few tens of nanometers inat least one dimension. Well-known examples include most clays and metal (oxyhydr)oxides (with ferrihydrite, an iron oxyhydroxide, as a type example).
- Mineral nanoparticles are defined as minerals that have nano-dimensions, but these are minerals that can also exist in larger sizes.
- Amorphous nanoparticles are the same, except without atomic structural order.
- Thin Films and Coatings also occur on the nanoscale. These may be on the order from a few nanometers to a hundred nanometers in thickness, and may either be natural or engineered. Surface films and coatings commonly have compositions and structures that are not directly related to their underlying substrates. Thin films can be characterized by AFM imaging, and by a variety of analytical methods such as Auger Electron Spectroscopy, X-ray photoelectron spectroscopy, and Time-of-Flight SIMS.
- Natural nanoparticles are ubiquitous in the Earth system. They have many sources; volcanic activity, cosmic dust, aeolian particles derived from weathering and wind erosion, chemical precipitation in many environments, biomineralization, and biomass combustion. Nanoparticles are important constituents of the atmosphere, oceans, soils, and in biota (produce or ingested by organisms). Detailed examples of Nanoparticles in the Earth system can be found on the related webpage on Nanoscience Topics in the Earth and Environmental Sciences.
Shapes of Nanoparticles
Hochella et al., (2008) Nanominerals, mineral nanoparticles, and earth systems: Science, v. 319, no. 5870, p. 1631-1635, provide some useful definitions for the shapes of nanomaterials: Nanoparticles "are as small as ~1 nM and may range up to several tens of nanometers in at least one dimension".
- Nanosheets or nanofilms have one dimension in this size range; (e.g., clay minerals)
- Nanorods have two dimensions in this size range
- Nanoparticles have three dimensions in this size range
- Nanotubes are nanoscale materials that have a tube-like structure; e.g., carbon nanotubes in the accompanying figure.
New Understanding of the Nano-World: Contexts and Concepts
The nanoscience revolution requires a fundamentally new understanding of the properties of matter at the nanoscale. These concepts, their significance, and applications are explored in more detail throughout this website. Here are some contexts and concepts that require special consideration when dealing with the nanoscale compared with micro- or mesoscale:
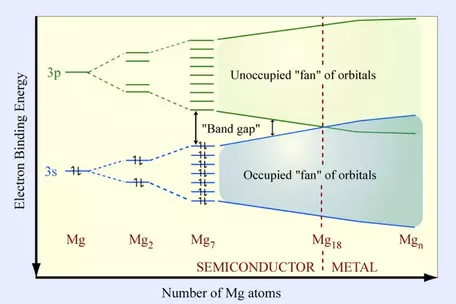
- Quantum effects are dominant on the nanoscale. An example of this can be seen in the accompanying figure where the "band gap" between S and P orbitals changes significantly as a function of the number of atoms involved, thus changing the electronic properties from a semi-conductor (<18 atoms) to metallic. See: Thomas, O. C., Zheng, W., Xu, S., & Bowen Jr, K. H. (2002). Onset of metallic behavior in magnesium clusters. Physical review letters, 89(21), 213403. Interatomic coulombic forces also become important in understanding the properties of matter. See for example: Zhang, H., and Banfield, J. F., 2014, Interatomic Coulombic interactions as the driving force for oriented attachment: CrystEngComm, v. 16, no. 8, p. 1568-1578.
- Properties of matter change as a function of particle size on the nanoscale. "When particle size is made to be nanoscale, properties such as melting point, fluorescence, electrical conductivity, magnetic permeability, and chemical reactivity change as a function of the size of the particle", (From Nano.gov).
- "Mineral nanoparticles also behave differently than larger micro- and macroscopic crystals of the same mineral. This observation violates aspects of the long-standing, formal definition of a mineral. Although definitions vary somewhat, depending on the source, the general consensus is that minerals are naturally occurring, crystalline substances having a characteristic and defined chemical composition (or compositional range in the case of solid solutions). For any particular composition, each mineral expresses a set of specific physical and chemical properties. Nanominerals and mineral nanoparticles satisfy these criteria, except that even with a fixed composition, they express a range of physical and chemical properties depending on their size and shape". Hochella et al., (2008) Nanominerals, mineral nanoparticles, and earth systems: Science, v. 319, no. 5870, p. 1631-1635
- Surface areas become quite large with respect to volume at the nanoscale.
- Consequently, surface energies become quite large with respect to Gibbs Free Energy. Surface energies are mostly ignored in conventional thermodynamic studies of phase relations. See the pioneering work of Alex Navrotsky and her colleagues on the thermodynamics of nanoparticles. Nanoparticles contribute substantially to the overall energetics of a system, but this contribution is often overlooked.
- Classical theory of nucleation and growth of crystals (one atom at a time into an ordered crystal structure) is not entirely accurate. Modern understanding of crystal growth indicates that crystal growth most commonly occurs by oriented aggregation of nanoparticles. See the recent work by DeYoreo, Carabello, Penn and Banfield among many others.
- Control of Grain Size on Solubility on the Nanoscale: One of the most important properties of nanominerals is solubility. The size effect on dissolution has long been described by this modified version of Kelvin equation. S0 is the solubility of the bulk material. It is typically measured in conventional dissolution studies. S is the solubility of exceedingly fine particles. This equation indicates that, as the particle size decreases, the solubility is expected to exponentially increase. This plot shows the ratio of S to S0 vs particle radius for quartz grains. It was calculated according to this equation using the parameters of quartz grains. You can see that when the size is larger than 10-7 m that is 100nm, this ratio equals 1. Nothing changes with the size. Only when the size goes down to this nanoscale, the ratio substantially deviates from 1 and the size effect on solubility can be observed. However, this equation was proposed based on theoretic calculation. Very few experimental studies have been reported to support it. Image and explanation from Michael Hochella, Virginia Tech.
- The contribution of nanoparticles to global bio/geochemical cycling is rarely considered. The global budget for naturally occurring inorganic nanoparticles. All numbers are in units of terragrams (Tg = 1012 g). All italicized numbers are fluxes (Tg yr-1), and the numbers in rectangular boxes are reservoir sizes, if known. Some of the nanomaterial fluxes are listed as two components, explained as follows: for the volcanic input to the atmosphere, 20 Tg is due to SO2 aerosol formation, and 2 Tg is due to mineral ash. For the three aeolian inputs to the continents, continental shelves, and the open oceans, the first number is due to the 320 Tg continental mineral dust output, and the second number is due to the 22 Tg volcanic output. Image and explanation from Michael Hochella, Virginia Tech.
Processes that Occur on the Nanoscale
The interfaces between material surfaces and their environment at the atomic scale is where all the (chemical) action takes place! Some of the surface-mediated reactions that affect the rates and pathways of Earth processes and global biogeochemical cycling include:
- Dissolution/Precipitation Reactions
- Catalytic Reactions
- Sorption Reactions
- Redox Reactions
Size Dependent Properties
Size Dependent Optical Properties
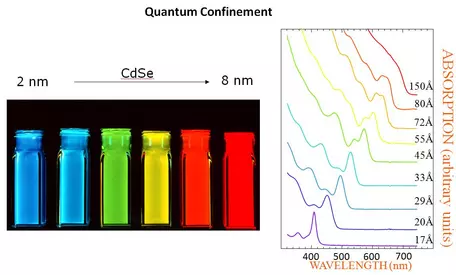
See the online presentation on Quantum Dots by Gerhard Klimeck, posted on nanoHUB, and Amiri et al., 2013, Preparation and Optical Properties Assessment of CdSe Quantum Dots. Materials Sciences and Applications, vol 4, p. 134-137.
Gold and silver nanoparticles also show size-dependence in their optical properties. An example of changing optical properties (color) as related to nanoparticle shape (prisms v. spheres) and size can be found at Dr. Shengli Zou's (Chemistry, University of Central Florida) website on Optical properties of nanoparticles and their applications. An explanation of this phenomenon, from the nanoComposix website on Nanoparticles: Optical Properties: "Gold nanoparticles absorb and scatter light with extraordinary efficiency. Their strong interaction with light occurs because the conduction electrons on the metal surface undergo a collective oscillation when they are excited by light at specific wavelengths. This oscillation is known as a surface plasmon resonance (SPR), and it causes the absorption and scattering intensities of gold nanoparticles to be much higher than identically sized non-plasmonic nanoparticles. Gold nanoparticle absorption and scattering properties can be tuned by controlling the particle size, shape, and the local refractive index near the particle surface". An example of the application of Au nanoparticles to biomedicine can be found in the article Gold and Silver Nanoparticles: Synthesis Methods, Characterization Routes and Applications towards Drugs byDhalid Alaquad and Tawfik Saleh, 2016, Journal of Environmental and Analytical Toxicology, 6:384. doi:10.4172/2161-0525.1000384
Size Dependent Crystallization
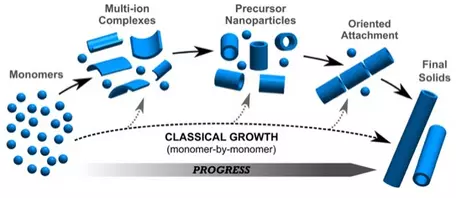
Size Dependent Dissolution
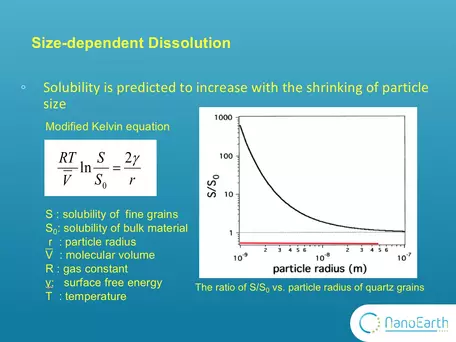
`ln(p/p_0) = (2gammaV_m)/(rRT)`
where p is the actual vapour pressure, p0 is the saturated vapour pressure, γ is the surface tension, Vm is the molar volume of the liquid, R is the universal gas constant, r is the radius of the droplet, and T is temperature. In the diagram, So is the solubility of the bulk (macro) material and can be substituted for Po. It is typically measured in conventional dissolution studies. S is the solubility of fine (nano-scale) particles and can be substituted for P. This equation indicates that, as the particle size decreases, the solubility is expected to exponentially increase. This plot shows the ratio of S to So vs particle radius for quartz grains. It was calculated according to this equation using the parameters of quartz grains. You can see that when the size is larger than 10-7 m that is 100nm, this ratio equals ~1. Nothing changes with the size. Only when the size goes down to the nanoscale, the ratio substantially deviates from 1 and the size effect on solubility can be observed. However, this equation was proposed based on theoretic calculation. Very few experimental studies have been reported to support it. The image and explanation was contributed by Michael Hochella, Virginia Tech, NanoEarth NNCI project.
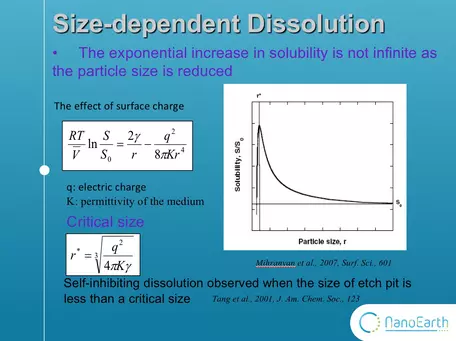
Here are some examples of size-dependent dissolution reactions at the nanoscale:
- Schmidt, J., and Vogelsberger, W. (2006). Dissolution kinetics of titanium dioxide nanoparticles: The observation of an unusual kinetic size effect, J. Phys. Chem. B, 110, pp. 3955–3963.
- Roelofs, F., and Vogelsberger, W. (2004). Dissolution kinetics of synthetic amorphous silica in biological-like media and its theoretical description, J. Phys. Chem. B, 108, pp. 11308–11316.
- Yang, Z. H., and Xie, C. S. (2006). Zn2+ release from zinc and zinc oxide particles in simulated uterine solution, Colloids Surf. B, 47, pp. 140–145.
- Liu, J., Aruguete, D. A., Jinschek, J. R., Rimstidt, J. D., and Hochella, M. F. (2008). The non-oxidative dissolution of galena nanocrystals: Insights into mineral dissolution rates as a function of grain size, shape, and aggregation state, Geochim. Cosmochim. Ac., 72, pp. 5984–5996.
- Erbs, J. J., Gilbert, B., and Penn, R. L. (2008). Influence of size on reductive dissolution of six-line ferrihydrite, J. Phys. Chem. C, 112, pp. 12127–12133.
- Cwiertny, D. M., Hunter, G. J., Pettibone, J. M., Scherer, M. M., and Grassian, V. H. (2009). Surface chemistry and dissolution of alpha-FeOOH nanorods and microrods: environmental implications of size-dependent interactions with oxalate, J. Phys. Chem. C, 113, pp. 2175–2186.
- Rubasinghege, G., Lentz, R. W., Park, H., Scherer, M. M., and Grassian, V. H. (2010). Nanorod dissolution quenched in the aggregated state, Langmuir, 26, pp. 1524–1527.
We have a lot to learn about the solubility of particles at the nanoscale!
Multimedia Resources that Introduce Nanoscience
- Nanotechnology: Super Small Science--from NBCLearn. Includes modules on: