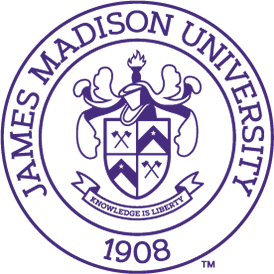
Laser spectroscopy of atmospherically relevant molecules and clusters in helium nanodroplets
Paul Raston, James Madison University
Location: Virginia
Abstract
Superfluid helium nanodroplets present an ideal medium for the study of chemical dynamics at the molecular level. Their low temperature, enormous heat conductivity, and weakly interacting nature allow for the investigation of various phenomena, such as how molecular rotation is effected by a solvent, and how molecules interact with each other. These two topics will be addressed in the lab by (1) measuring the spectra of unexplored molecules in helium nanodroplets and determining their rotational constants; this data will then be used to test known models describing the interaction between the molecule and helium solvent, and (2) synthesizing and characterizing unexplored molecular clusters in an effort to better understand molecular solvation; students will solvate the "unexplored molecule" with an atmospherically relevant species (O2, N2, H2O), and investigate the resulting clusters with laser spectroscopy.
Student Goals
- Select species that may yield interesting results and make predictions as to the outcome of the experiments. Then formulate a protocol, thus allowing for efficient experimentation.
- Explain and defend decisions during experimentation: For example, if expected outcomes are not met (like no signal), make well-reasoned (defensible) change(s) and repeat...
- Analyze and interpret data, then communicate research results.
Research Goals
- To identify and spectroscopically characterize an unexplored atmospherically relevant small molecule in superfluid helium nanodroplets.
- Using laser spectroscopy and electronic structure calculations, to investigate how that molecule interacts with another atmospherically relevant molecule (such as molecular oxygen, nitrogen, or water).
Context
There are two phases to this CURE. The first will take place in Physical chemistry II lab, where a team of students will work towards collecting and analyzing the infrared spectra of an unexplored molecule in superfluid helium nanodroplets (research goal 1). This should take 3-4 weeks to complete, with 5 hours of contact time (lab and "lecture") per week. The second phase will take place during the summer, where 2+ research students will explore the solvation of the molecule with an atmospheric species (research goal 2). For this phase, one student will perform the experiments, and the other will perform calculations. This should take 5-10 weeks of full-time (40 hrs/wk) research to complete.
Prior to the first leg of the CURE, the students need to have successfully completed an activity on the gas phase infrared spectroscopy of small molecules. For the second leg, students need to scrutinize the results from the first leg (lab notebook and report).
Target Audience:Major, Non-major, Upper Division
CURE Duration: A few class periods, Multiple terms
CURE Design
Recently we introduced the classic infrared spectroscopy of OCS in helium nanodroplet experiment in our physical chemistry II lab. Based on the positive feedback we decided to make the experience (potentially) more exciting by investigating an unexplored molecule. From the analysis and comparison with previous investigations, we hope to gain an improved understanding of how molecular rotation is affected by the presence of the helium solvent. Summer research students will take the results from this investigation and build off from it; students will synthesize and characterize unexplored molecular clusters in an effort to better understand molecular solvation.
Core Competencies: Analyzing and interpreting data, Asking questions (for science) and defining problems (for engineering), Constructing explanations (for science) and designing solutions (for engineering), Developing and using models, Planning and carrying out investigations, Using mathematics and computational thinking
Nature of Research:Basic Research
Tasks that Align Student and Research Goals
Student Goals ↓
Summarize literature on small molecules that have been investigated in helium nanodroplets.
Choose a small atmospherically relevant molecule to investigate, that has a large transition dipole moment in the range of an available laser.
Visually depict (1) the moment of inertia of both the molecule you choose and non-superfluid helium, and (2) the vibrational shift against the dipole moment difference.
Create a simulated spectrum of your molecule.
Outline a timeline for the experiment, based on well-reasoned experimental parameters.
Summarize literature covering the solvation of small molecular clusters in helium nanodroplets.
Visually depict their vibrational shift as a function of cluster size.
Predict the vibrational shift(s) of the cluster(s) under investigation.
Develop a timeline for the experiment.
Choose an appropriate computational methodology to predict vibrational shift.
Briefly summarize the status of the experiment (is it working? How do you know?).
Propose and defend the next step in the experiment (do changes need to be made?).
Gather feedback from others (peers and instructor) about proposed experimental decisions (molecule of choice, experimental parameters...).
Summarize the status of the experiment.
Propose and defend the next step in the experiment.
Gather feedback from others.
Use your favorite spectral fitting program (e.g. PGOPHER of SPFIT) to reduce the spectrum to a set of molecular constants!
Visually depict how they compare with those previously determined (in the literature).
Present your research to the class (15 min. talk).
In your designated groups, write a paper about the research; indicate who did what.
Compare calculated and measured vibrational shifts.
What is the structure?
Why is the structure what it is?
Present your research to the class.
Write a paper about the research.
Instructional Materials
N/A
Assessment
Lab reports; team presentations; classroom discussions.
Instructional Staffing
Paul Raston is the laboratory coordinator.
Chakree Tanjaroon is an instrument chemist who has expertise in molecular spectroscopy.
Author Experience
Paul Raston, James Madison University
My motivation is to provide an opportunity for students to experience fundamental physical chemistry research using a sophisticated apparatus that is otherwise only accessible at schools with graduate programs.
Advice for Implementation
Have a working spectrometer (see following)!
Iteration
The most challenging aspect of implementing this CURE was having the helium nanodroplet isolation spectrometer functional. Due to a series of equipment failures, students were only able to perform a single experiment in the first iteration of this CURE. The experiment involved measuring the spectrum of 13C labelled formic acid in helium nanodroplets (for the first time), which did indeed open new pathways for research that students will investigate in the future.
Using CURE Data
The student acquired digital research data will be saved as ".dat" (ASCII) file type, so that they can be broadly accessed. The instructors will take primary responsibility for the storage of CURE data.
Resources
[1] J. P. Toennies, and A. F. Vilesov, Angewandte Chemie International Edition, 43, 2622 (2004).
[2] F. Stienkemeier, and K. K. Lehmann, Journal of Physics B: Atomic, Molecular and Optical Physics, 39, R127 (2006).
[3] M. Y. Choi, G. E. Douberly, T. M. Falconer, W. K. Lewis, C. M. Lindsay, J. M. Merritt, P. L. Stiles, and R. E. Miller, International Reviews in Physical Chemistry, 25, 15 (2006).