Part 5—Map and Interpret Regional Motion
Step 1 – Plot Your Velocity Vector(s) on a Map
- To plot your vector, you'll access a base map that shows the locations of the GPS stations. Select (highlight) and Copy the following line of text:
http://www.unavco.org/cws/modules/pnw_eo.kml - Go to Google Maps (will open in a new window).
- Paste the text you copied above into the "Search the map" field (choose Edit > Paste) then click the Search Maps button.
- Click My Maps, then click Create new map.
- Google Maps requires users to have a Google Account to use their services. If you already have an account, sign in.
- Give your map a name and decide if you want it to be Public or Unlisted. Click the UNAVCO Permanent Stations checkbox and expand the selections by clicking the plus sign to find the station for which you produced the annual velocity vector.
- Select the Draw a line tool from the Google Drawing Tools icon and click once on the location that represents your GPS station. Note that as you move your cursor away from the start point, the length of your line is shown on the screen. To finish a line, double-click where you want it to end. Draw a few practice lines so you are comfortable with the tool, then delete them.
- A convenient scale to use for drawing velocity vectors is 1 mm/year = 10 miles. Thus, a rate of 6.9 mm/year would have a vector with a length of 69 miles. Note the length (in miles) of the vector you are plotting.
- To reproduce your vector at the correct angle from north that you determined earlier in Part 4Calculate Station Motion, hold a protractor up to your screen, or open an online protractor in another window.
- Starting at the station point you have selected, draw your vector using a straight line for the distance and angle you have determined.
- Select the Draw a shape tool from the Google Drawing Tools icon and practice drawing a few very small triangles. Delete them after you are familiar with how the tool works. Note that you can only move one point at a time after a shape is drawn. You will need to draw a triangle to complete the "arrow" end of your vector.
- Complete your vector by drawing a triangle whose point is on the extreme end of the line you drew (away from the station where you started). Make sure that the arrow head does not make your vector longer than the line you drew.
Step 2 – Interpret Motion and Deformation from Vectors
Compile several velocity vectors in the region onto a single map. Even if you are working alone, you can download data and produce velocity vectors for a few additional stations fairly quicklyyou'll likely be surprised at how much more easily and quickly you can complete the task the second and third times you do it!
Examine the pattern of velocity vectors on your map. Consider what the differences in the vectors indicate about that portion of the North American plate.
- Should the North American plate be considered "rigid"?
- Which portions of the plate would you expect to be experiencing the most intense deformation and regional metamorphism?
- Is there a relationship between the magnitude of the velocity vectors and their geographic locations (coastal, urban corridor, or Eastern)?
- What possible outcomes can you imagine if different portions of the plate continue moving at different rates over a million years?
Step 3 – Consider Other Geologic Processes and Features in the Region
Plate tectonic researchers make and study maps that are similar to the one you produced. Automated data processing and mapping functions allow them to plot and view velocity vectors for many GPS stations at once.
- Show and examine the map below. Revisit the Talk About It questions from above in light of additional information on the map.
- What other data would you need to interpret the geologic setting of the region?
- How does the location of earthquakes and volcanoes complement the story revealed by the velocity vectors?
- What relationships do you think might exist among earthquakes, volcanoes, and motion?
- Can you detect a relationship between the locations of earthquakes and the velocity of the GPS stations?
- Describe how the information on the final map refines your mental model of processes near the Cascadia Subduction Zone.
Use the additional information below to help integrate your knowledge of station motion, seismic hazards, and volcanic hazards.
Cascadia Subduction Zone Seismic Hazards
Three types of earthquakes are associated with the CSZ: large subduction zone earthquakes, small to moderate deep earthquakes in the Juan de Fuca plate, and small to moderate shallow earthquakes in the North American plate. Subduction zone earthquakes occur on the plate interface between the subducting plate and the overlying plate. The top of the subduction zone can remain locked for years, before slipping during magnitude 9+ megaquakes. The last subduction zone earthquake was in 1700. This earthquake was hypothesized by Brian Atwater of the United States Geological Survey. He studied Japanese paintings, writings, records, and Native American oral histories to piece together the records of a tsunami that struck Japan and the Pacific Northwest in 1700. He traced the evidence back to a large earthquake on the Cascadia Subduction Zone.
Because subduction zone earthquakes have the greatest magnitudes and are the most likely to be accompanied by a tsunami, they are the most dangerous hazard for the Pacific Northwest. Significant hazards are also associated with deep and shallow earthquakes, though these are not as catastrophic.
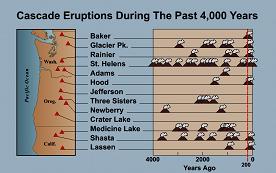
Volcanic Hazards
A line of active volcanoes called the Cascades parallels the coast of the Pacific Northwest. Volcanic chains such as this one commonly form above subduction zones: as a plate plunges into the mantle, higher temperatures and pressures begin to partially melt the plate at about 100 km depth. These conditions cause the plate to "sweat" out volatiles, and these enhance melting of the surrounding materials. The melted materialmagmarises to the surface, resulting in a line of volcanoes parallel to the offshore trench where one plate moves beneath the other.
The last major eruption in the Cascades volcanoes was Mount St. Helens in 1980. Mount St. Helens is not the only active Cascade volcano; all of the Cascade volcanoes are capable of having a major eruption. There is significant risk associated with a volcanic eruption, so careful monitoring is necessary. Both the United States Geological Survey (USGS) and EarthScope have installed instrumentation on the Cascade volcanoes.
The concept that Earth's crust consists of rigid plates that move relative to one another is a unifying theory that connects many seemingly unrelated geologic phenomena. These include the location of linear mountain chains, volcanoes, earthquakes, and deep-sea spreading ridges. The new continuous high-precision PBO data are allowing scientists to further refine the plate tectonic model, and test hypotheses concerning the nature of boundary zones and the rigidity of plates. For example, as the Juan de Fuca plate subducts under the North American plate, it does so at an angle to the trench (subduction is to the northeast, while the trench is roughly oriented north-south) and rotates the upper-plate, breaking it up into smaller rotating blocks. In addition, Oregon is squeezing against Washington, which is responsible for upper plate shallow earthquakes and faults, such as the 2004 Nisqually earthquake.