Volunteered Abstracts and Poster Session
LA RISTRA: Lithospheric and upper mantle structure of the Rio Grande Rift
Rick Aster, New Mexico Institute of Mining and Technology
Jim Ni, Dave Wilson, Wei Gao, Steve Grand, W. Scott Baldridge, Steve Semken and Michael West
download poster file (PowerPoint 3MB Oct11 05)
Is the Arc Accretion Model for the Crustal Evolution of Southern Laurentia Correct? Let's Look at the Rocks!
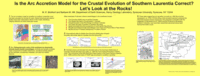
download poster file (Acrobat (PDF) 4.1MB Oct21 05)
During the last 20 years the prevailing wisdom has been that southern Laurentia evolved by accretion of successively younger island arc terranes during the interval 1780-1650 Ma. Indeed, the results of recent seismic studies have been interpreted in terms of this model, with dipping reflectors identified as fossil subduction zones. However, Paleoproterozoic rocks of the southwestern United States are dominantly bimodal metavolcanic assemblages and related granitoids. Examples include the Gunnison-Salida region of central Colorado, where metavolcanic assemblages consist of pillowed tholeiitic basalt and high-silica rhyolite, bimodal assemblages in the Needle Mountains in southwestern Colorado, and both basalt-dominated and rhyolite-dominated bimodal assemblages in New Mexico. Although some intermediate volcanic rocks occur in the Paleoproterozoic of Arizona, bimodal assemblages are also dominant there. In the Paleoproterozoic of the southwest, andesite and basaltic andesite, major components of modern island arcs, are almost entirely absent, as are ophiolites, accretionary m途Early Tertiary compression and subsequent relaxation, on the Proterozoic Belt basin, and on Archean terrane boundaries have not been completely deciphered. Each of these major tectonic features influenced the architecture of the crust. Only a comprehensive geological effort, involving detailed mapping, geophysical imaging, geochronological constraints and geochemical studies can bring us a clearer understanding of crustal structure in western Montana.
The Lowland Creek volcanics erupted in western Montana between 53—48 Ma (all dates are 39Ar/40Ar determinations done by V. Ispolatov and L. Snee). The volcanic field lies astride the proposed Great Falls tectonic zone, and is elongated in a NE—SW direction. Two rhyolitic tuff units, at 53???0.2 Ma and 51.6???0.2 Ma, comprise the bulk of the stratigraphy, and approach 600 m in thickness. The younger tuff lies on the west side, whereas the older one covers most of the eastern half of the field. Intrusive dacitic and rhyolitic plugs are the youngest units. The Lowland Creek is restricted to compositions more silicic than andesite (~ 60 wt. % SiO2). Xenocrystic plagioclase, garnet, and possibly amphibole, major element chemistry, and the Sr, Nd and Pb isotopic compositions of the volcanics all indicate that middle or lower crustal protoliths were the major sources of the magmas. In the western end of the field, there is permissive Nd isotopic evidence that juvenile, mid-Proterozoic crust was a significant source material. These observations suggest that the intrusive units of the Lowland Creek can be used to map the subsurface location of the Archean—Proterozoic boundary along the projected trace of the Great Falls tectonic zone. The Lowland Creek volcanics may pre-date or be contemporaneous with the Anaconda core complex, though the timing of its development is as yet poorly constrained. It is unclear whether generation of Lowland Creek magmas was linked with decompression during core complex formation. Tilting of the Lowland Creek tuff units near Butte demonstrates that faulting and tilting of the 65—63 Ma ore deposits in the Butte district occurred after or during formation of the Lowland Creek field.
Figure: Sieve-textured, xenocrystic plagioclase (~An30) is overgrown by plagioclase that is in equilibrium with the dacitic host lava (~An50). Sample LCV 19-3, crossed polars, field of view ~ 2 mm.Overview of the Clark Fork Watershed Education Program (CFWEP)
Colleen Elliot, Montana TechCFWEP began as a pilot program in three Butte schools in 2003. We are now funded to expand to 31 schools in four counties from Butte to Bonner. CFWEP is dedicated to using outdoor activities and local scientists and engineers to teach both educators and sixth through 12th grade students the scientific principles and methods for studying their watershed. Our philosophy is that place-based, hands-on, field and classroom activities instill in participants a clearer grasp of fundamental science concepts and procedures as well as a sense of watershed stewardship.
The Clark Fork River links the largest contiguous complex of Superfund sites in the United States. The region was designated a Superfund site on the National Priorities List in the early 1980s on the basis of damages caused by almost 150 years of copper mining and smelting operations in Butte and Anaconda. Ecosystems and communities of the entire basin have been heavily impacted by mining wastes disposed in and transported via Silver Bow Creek and the Clark Fork River, as well as by airborne effluents primarily from the historic Washoe smelter in Anaconda.
Our project addresses three specific needs within the Upper Clark Fork watershed: The first is for citizens who have the scientific background to understand how natural systems work; The second is for citizens who understand what a healthy natural environment is and who have a sense of stewardship that leads them to establish and maintain one here; The third need is for ongoing and continuous monitoring of riparian health as the basin undergoes one of the biggest science experiments of all times. Over the next few decades, hundreds of millions of dollars will be spent to restore Silver Bow Creek and the Upper Clark Fork River to a fully functioning watershed. Our students will be an integral, dynamic part of this process.
The primary goals of CFWEP are accomplished by the relationships formed through pairing professional scientists and engineers who work in the Upper Clark Fork Watershed with students and their teachers. Through this relationship, participants will gain a working knowledge of the geology and biology of the Clark Fork watershed. Using first-hand knowledge and skills gained through their relationships with scientists and field training workshops provided by CFWEP, participating teachers will have the ability to create and implement teaching plans that meet CFWEP's goals as well as state and national education standards.
EarthScope resources will expand the scientific scope of our program and enhance student research projects within the upper Clark Fork basin. Students and teachers are already studying sediment transport and deposition with the basin. EarthScope data and scientists can help students tackle larger questions related to the tectonic geomorphology of the northern Rocky Mountains, and help explain, for instance, why the Big Hole and Deer Lodge Valleys are so deep, and how they evolved.
CFWEP is housed in the Technical Outreach Department of Montana Tech of the University of Montana, and has three full-time staff members:
Colleen Elliott, Program Administrator
Matt Vincent, Science Coordinator
Kyle Gunderman, Grant Writer
Has the Wyoming Craton lost its keel?
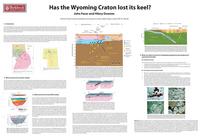
download poster file (Acrobat (PDF) 3.1MB Oct11 05)
References: [1] Gorman et al.(2002) Can.J.Earth Sci.39, 375-398; [2] Davis et al. (1995) Lithoprobe Report 47, 329-334; [3] Irving et al.(1997) EOS, 78., F786; [4] O'Neill & Lopez (1985) AAPG, 69, 437-447; [5] Collerson et al. (1993) IGCP Project 304, International Workshop, Macquarie University, Sydney, 2-3 October 1993; [6] Carlson et al. (1999) Proc. 7th International Kimberlite Conf. 353-360; [7] Carlson et al. (2004) Lithos, 77, 453-472; [8] Downes et al.(2004) J. Petrology, 45, 1631-1662.
A Geologic-GIS Database for the Yellowstone-Teton Volcanic and Tectonic Region

download poster file
Origin and tectonic implications of across strike geochemical variations in the Eocene Absaroka Volcanic Province, USA
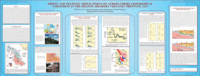
Todd C. Feeley, Montana State University
download poster file (PowerPoint 740kB Oct11 05)
Proterozoic Evolution of the Western Margin of the Wyoming Craton: Implications for the Tectonic and Magmatic Evolution of the Northern Rockies
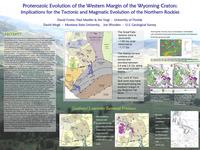
David A. Foster, Paul A. Mueller, University of Florida
David W. Mogk, Montana State University
James Vogl, University of Florida
Joseph Wooden, US Geological Survey
download poster file (PowerPoint 4.5MB Oct11 05)
A Transect Across Alaska, from Pacific to Arctic Margins

download poster file (Acrobat (PDF) 801kB Oct20 05)
During the period 1984 to 1990, the U.S. Geological Survey, University collaborators, and the Alaska Division of Geological and Geophysical Surveys investigated the crust of Alaska along a ~1500-km-long, north-south corridor. The project, known as the Trans-Alaska Crustal Transect (TACT), extended from the trench in the Gulf of Alaska to the Arctic coast. The data collected included refraction/wide-angle reflection, vertical-incidence reflection, geologic, gravity, magnetic, magnetotelluric, earthquake, and petrophysical data. In most places onshore, the seismic data were collected along or near the Trans-Alaska Oil Pipeline. The transect crossed oceanic, oceanic-arc and platform, and continental-marginal terranes that have been variously subcreted, accreted, rotated, imbricated by thrust faults, translated by strike-slip faults, and intruded by numerous magmatic arcs. We use the data collectively to present an interpretation of the structure and tectonic history of Alaska. Currently in the Gulf of Alaska, the Pacific plate and the overlying Yakutat terrane are subducting beneath and colliding with the North American plate. (The Yakutat terrane includes fragments of an accretionary prism and of the Pacific plate.) Subduction is resisted by the buoyancy of this doubly thick oceanic crust. Currently on the Arctic margin of northeastern Alaska, there is compression that may be related to the ongoing subduction/collision of the Pacific plate/Yakutat terrane (PAC/YAK) in the south.
The most spectacular structures and the deepest Moho along the transect are located near the Pacific and Arctic margins. Near the Pacific margin, the Chugach Mountains are being uplifted by the subduction/collision of the PAC/YAK and are underlain by a stack of subcreted oceanic layers similar to the PAC/YAK. We interpret this stack as remnants of the extinct Kula (or Resurrection) plate that was converging rapidly on North America in the latest Cretaceous and early Cenozoic. Continental Moho just landward of this subcreted stack is more than 55 km deep, in an area of low-lying topography. Near the Arctic margin, the Brooks Range and southern part of the North Slope are underlain by striking duplex structures that extend ever deeper into the crust from north to south. We interpret these duplexes to overlie a crustal-scale wedge consisting of continental crust of the North Slope passive margin and an attached fragment of mantle that has moved southward with respect to the North American plate. Moho has been depressed to nearly 50-km depth beneath the crest of the Brooks Range. The tectonic history leading to the current structure of northeastern Alaska is complex, with several compressional episodes and at least one extensional episode occurring between Late Jurassic/Early Cretaceous and the present.
Central Alaska consists of continental-marginal terranes and at least one oceanic arc that collided with these terranes in the Late Jurassic/Early Cretaceous and was partially obducted. Some of these terranes were oroclinally folded in the Early Cretaceous, and many were extended and intruded in the mid-Cretaceous. Offset along major strike-slip faults (Tintina and Denali faults) followed. Moho is everywhere between 30 and 35 km deep in central Alaska.
New 40Ar/ 39Ar dates from alkaline intrusions in the northern Crazy Mountains, Montana: Implications for the timing and duration of alkaline magmatism in the Central Montana Alkalic Province
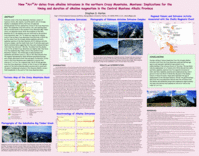
download poster file (Acrobat (PDF) 1.4MB Jun2 06)
Paleomagnetism and 40Ar/39Ar Dating of Folded Sills in the Lombard Thrust Sheet, South Central Montana: Implications for the Timing of Fold and Thrust Deformation and Vertical Axis Rotations along the Southern Margin of the Helena Salient
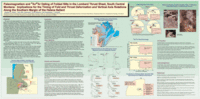
John Wm. Geissman, University of New Mexico
Chris Whisner, University of Tennessee
Christopher J. Schmidt, Western Michigan University
download poster file (Acrobat (PDF) 629kB Nov3 05)
40Ar/39Ar dates on biotites from sills from two sills in two different folds yield identical plateau dates of 77.18 ??? 0.31 Ma and 77.00 ??? 0.31 Ma (2), consistent with a Late Cretaceous age for deformation.
Although the group mean directions from each of the four folds probably represent spot readings of the geomagnetic field and the number of spot readings is low, their structurally corrected grand-mean direction (Dec.=344°, Inc.= 65°, k= 82, 95= 10°, N= 4) is statistically indistinguishable from the expected Late Cretaceous cratonic reference direction from the Adel Mountain Volcanics of western Montana. Consequently, these results suggest that hanging wall strata in the Lombard Thrust Sheet of the Helena Salient in this vicinity have probably not experienced significant local vertical axis rotation ( R= -8.5° ??? 20.5°) due to thrust movement along the Southwest Montana Transverse Zone.
Geochronological Evidence for Meso- And Neoproterozoic Mafic Magmatism along the Western Margin of the Wyoming Craton
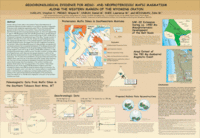
Wayne R. Premo, Daniel M. Unruh, Lawrence W. Snee, US Geological Survey
John W. Geissman, University of New Mexico
download poster file (Acrobat (PDF) 238kB Nov3 05)
Cenozoic Sedimentary and Tectonic Evolution of the Flint Creek Basin, West-Central Montana
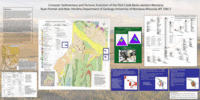
download poster file
Sedimentary basins commonly represent the best preserved and highest resolution record of tectonic history in areas undergoing active deformation. In this poster, we present an example from recent work we have conducted in the Flint Creek basin of west central Montana. The Flint Creek basin provides a record of deformation and landscape evolution in the region from middle Eocene time through late Miocene time. Transtensional stress along the Lewis and Clark Lineament to the north and rapid slip with in the Anaconda metamorphic core complex to the south, were synchronous with initiation of Flint Creek basin subsidence and local volcanic outpourings during the middle to late Eocene.
Existing faunal assemblages collected from Tertiary strata above basal volcanic rocks in the basin indicate a late Oligocene to late Miocene age and correlate with the Bozeman Group of southwest Montana. Paleosol characteristics, clay mineralogies and faunal assemblages of these strata suggest a subhumid to semiarid paleoclimate. Sediments of the upper Renova Formation (Cabbage Patch beds) were deposited in alluvial, lacustrine and palustrine environments. Paleocurrent indicators and petrographic analyses of Cabbage Patch sandstones imply a northwesterly paleoflow of arkosic detritus during the late Oligocene (Arikareean North American Land Mammal Age). Exhumation of the Anaconda metamorphic core complex to the south of the Flint Creek basin would have been a primary source for the 2-mica rich feldspathic sand characteristic of Cabbage Patch sandstone facies. Volcanic detritus common to these sandstone facies was likely derived from denudation of the Elkhorn Mountain and Lowland Creek volcanic edifices, which both overlie the Boulder batholith to the southeast.
Excellent exposure of a localized 2-meter thick boulder bed with underlying smectitic clay and barite nodules represents the regionally extensive mid- Miocene unconformity (Hemingfordian North American Land Mammal Age). Massive siltstone, calcrete and gravel facies of the lower Sixmile Creek Formation (Flint Creek beds) overlies the unconformity. The Flint Creek beds were subsequently tilted and eroded some time during the middle to late Miocene when last movement on intrabasinal normal faults occurred. Sand and gravel facies typical of the upper Sixmile Creek Formation (Barnes Creek beds) were deposited in a paleovalley eroded into underlying strata. A large alluvial fan bajada complex shed detritus northward from the uplifting Flint Creek and Sapphire ranges into the axial Barnes Creek fluvial system.
The middle crust of the Wyoming Province: ground-truthing above 2000 meters elevation in the Beartooth Mountains, Montana and Wyoming
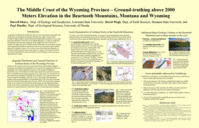
Darrell J. Henry, Louisiana State University
David W. Mogk, Montana State University
Paul A., Mueller, University of Florida
download poster file (PowerPoint 3.5MB Oct11 05)
Archean rocks are exposed in the eastern and central Beartooth Mountains, Bighorn Mountains, and samples from deep-drill cores in eastern Wyoming and Montana are dominantly Late Archean granitoids, members of the tonalite-trondhjemite-granodiorite suite, with inclusions of older supracrustal rocks preserved as tectonic slices or pendants in the younger magmatic rocks. These are considered to be part of the Beartooth-Bighorn Magmatic Zone (BBMG). The Archean rocks in the western Beartooth Mountains and to the west are dominantly older high-grade gneisses with varying abundances of metasedimentary rocks. This area is generally termed the Montana Metasedimentary Province (MMP). The dominantly magmatic terrane in the eastern and central Beartooth Mountains and the high-grade gneiss terrane to the west are separated by a major discontinuity in the Archean basement marked by a mobile belt in the North Snowy Block, western Beartooth Mountains.
On a finer scale in the Beartooth Mountains we recognize four geographically and geologically distinct domains: the Beartooth massif, the Stillwater Block, the North Snowy Block and the South Snowy Block represent distinct lithologic, structural, and chronologic subdivisions. The main Beartooth massif consists predominantly of voluminous Late Archean igneous granitoids (2.8-2.9 Ga) with inclusions of metasupracrustal rocks, which exhibit wide ranges in sizes (cm to km), composition, metamorphic grade, and isotopic age (to 3.3 Ga). The peak metamorphic conditions likely associated with the early stages of granitic magmatism are considered to be 5-7 kbar and 750-800ºC. The North Snowy Block is interpreted as a collage of several allochthonous units structurally juxtaposed against the main Beartooth Massif with latest Archean magmatism suggesting this juxtaposition occurred about 2.55 Ga. The Stillwater Block is dominated by the mafic layered Stillwater Complex (2.7 Ga) and its contact aureole that developed in older metasedimentary rocks. The South Snowy Block is dominated by lower grade metasedimentary rocks and a series of locally important 2.7 Ga old granitoid plutons.
EarthScope could address several additional questions that are related to the Beartooth and other Wyoming Province uplifts: What is the character of the faulting at depth that brought these middle crustal blocks to the surface and what is the relation of these faults to Archean structures? What is the nature of the crust below the 3000 m of mid-crustal level lithologies currently exposed in the Beartooth Mountains? Are major subprovince boundaries (e.g., BBMZ-MMP) largely vertical or horizontal structures? Do any of the geologically recognizable province or sub-province boundaries extend to the mantle?
Tectonic and Thermal Architecture of the Sevier Orogen in Western Montana
Thomas J. Kalakay, Rocky Mountain CollegeDavid A. Foster, University of Florida
Jeff Lonn, Montana Bureau of Mines and Geology
Warren C. Grice Jr., University of Florida
I submit this so that this hypothesis will be remembered as EarthScope progresses eastward. I welcome comments at glkinsland@louisiana.edu.
The Big Faults in Northern Idaho and What We Know About Them
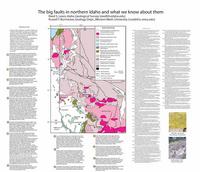
Russell F. Burmester, Western Washington University
download poster file (Acrobat (PDF) 917kB Oct10 05)
Faults at the surface in northern Idaho are mostly within Belt Supergroup strata and igneous rocks that intrude them. Many probably have long and complex histories that involve basement rocks and an accommodation of Belt basin subsidence. Some moved during Jurassic through Paleocene contraction, and were reactivated or newly formed during Eocene extension. From north to south, fifteen important faults are identified: (1) Moyie fault, a compressional structure within the Purcell anticlinorium; (2) Spokane dome mylonite zone, a thick tops-east zone that might be equivalent to the basal d鰰C/Ma), and subsequent variable Pb-loss in the apatite and rutile systems during a Mesozoic thermal perturbation to the deep lithosphere.
Closure of the rutile thermochronometer signals temperatures of less than or equal to 450°C in the lower crust during attainment of cratonic lithospheric conductive geotherms, and such closure in the examined portions of the "off-craton" Proterozoic domains of southern Africa indicates that their lithospheric thermal profiles were essentially cratonic from the Neoproterozoic through to the Late Jurassic. These results suggest similar lithospheric thickness and potential for diamond stability beneath both Proterozoic and Archean domains of southern Africa. Subsequent partial resetting of U-Pb rutile and apatite systematics in the cratonic margin lower crust records a transient Mesozoic thermal modification of the lithosphere, likely related to mantle upwelling and rifting of Gondwana during the Late Jurassic to Cretaceous. The manifestation of this thermal pulse in the lower crust is spatially and temporally correlated with anomalously elevated and/or kinked Cretaceous mantle paleogeotherms, and evidence for metasomatic modification in cratonic mantle peridotite suites. It is argued that most of the geographic differences in lithospheric thermal structure inferred from mantle xenolith thermobarometry are likewise due to the heterogeneous propagation of this broad upper mantle thermal anomaly (Bell et al., 2003). The differential manifestation of heating between cratonic margin and cratonic interior suggests the importance of advective heat transport along pre-existing lithosphere-scale discontinuities, and necessitate further consideration of advective heat transport mechanisms for the transient, and spatially heterogeneous elevation of Late Mesozoic thermal gradients throughout the southern African continent. This Mesozoic thermal and chemical modification of the lithospheric mantle may be a consistent feature of the Gondwanide continental fragments.
As the Earthscope geophysical facility moves across the Northern Rockies, opportunities to link the the present-day geophysical snapshot of lithospheric properties and structure to xenolith thermochronological studies will greatly enhance our ultimate understanding of the 4-D architecture and operative processes in the evolution of the North American continental lithosphere.
References:
Bell, D.R., Schmitz, M.D. and Janney, P.E., 2003, Mesozoic thermal evolution of the southern African mantle lithosphere: Lithos 71, 273-287.
Schmitz, M.D. and Bowring, S.A., 2003, Constraints on the thermal evolution of continental lithosphere from U-Pb accessory mineral thermochronology of lower crustal xenoliths, southern Africa: Contributions to Mineralogy and Petrology 144:592-618.
The 26 July 2005 Dillon, Montana earthquake
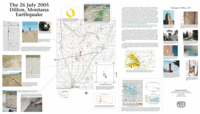
download poster file (Acrobat (PDF) 5.1MB Oct20 05)
Mapping the extent of the Archean craton in the deep crust of the Northwest U.S.
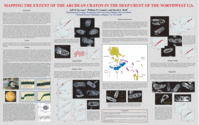
William P. Leeman, National Science Foundation
David E. Wolf, Washington State University
download poster file
Knowledge of the chronology and composition of the lithosphere of the northwest United States is fundamental to our understanding of the structure and evolution of the North American continent. Within a few hundred kilometers across W Montana to E Washington and E Oregon, the basement makes an abrupt transition from evolved Archean-Proterozoic crust of the North American craton to juvenile Mesozoic and Cenozoic crust. The details of the crustal architecture in much of this region, however, are poorly known due to complicated structural relationships, limited geochronological studies, and blanketing by recent volcanism. Xenoliths carried from the deep crust by recent eruptions provide us with an opportunity to examine the evolution of this otherwise inaccessible part of the North American lithosphere and map the western extent of the Archean craton. Laser ablation (LA) ICPMS U-Pb geochronology of zircons from these xenoliths has been an important method in this regard and has helped constrain the magmatic and tectonic evolution of the craton. Our new xenolith zircon ages show unequivocally that Archean basement is present further west than can be inferred from surface exposures.
The nature of the basement beneath much of southern Idaho is particularly poorly known due to limited surface exposures and an extensive blanket of Miocene-Recent Snake River Plain (SRP) rhyolite-basalt volcanism. Archean basement is exposed as far west as SW Montana, S-central Idaho and NW Utah. Its presence has been inferred beneath the eastern SRP from the occurrence of granulite xenoliths in some SRP lavas, but ages are based only on whole rock Pb-Pb and Sm-Nd isotopic data. To better constrain the age and composition of the basement beneath the SRP, and its possible influence on Phanerozoic magmatic and tectonic processes, we determined U-Pb zircon ages for four mafic to felsic granulite xenoliths carried by SRP lavas from three localities along its northern margin: Square Mountain in the west (DM103, opdalite, 73.8% SiO2), Craters of the Moon National Monument (COM9A, opdalite, 73.1% SiO2; COM22, enderbite, 68.5% SiO2), and Spencer/Kilgore volcanic field in the east (SK3, norite, 54.9% SiO2). These xenoliths have been subjected to granulite grade metamorphism with Ts>700-750°C as evidenced by anhydrous mineral assemblages and mineral thermometry (plag-san and/or ilm-mt). The host lavas for the xenoliths have ages of ca. 4 Ma, 2-10 Ka, and <10 Ka, respectively.
Zircons were separated from the xenoliths using standard procedures and analyzed by LA-ICPMS using a Nd-YAG 213nm laser and ThermoFinnigan??? Element2 HR-ICPMS at Washington State University. All xenoliths contain zircons yielding Archean ages, with a general trend of decreasing age from east to west. Most samples exhibit complex Archean histories with multiple age populations, possibly from different magmatic episodes: zircons from SK3 yield 207Pb/206Pb ages clustering around 3.10, 2.90 and 2.76 Ga; COM9A has ages clustering at 2.76 Ga and between 2.60 and 2.50 Ga; COM22 has a bimodal population with age peaks at 3.20 and 2.75 Ga, a scattering of ages between 3.2 and 2.75 Ga, and one older core > 3.5 Ga; DM103 zircons yield a consistent age of 2.58 Ga. Zircons from COM22 and SK3 have complex low-U overgrowths that yield recent 206Pb/238U ages.
This preliminary study reveals important information about the evolution of the lithosphere in southern Idaho. First, Archean basement extends throughout this region as evidenced by Archean ages in all of the samples. Second, all samples have lower concordia intercepts near 0 Ma and evidence for recent zircon growth, probably related to modern SRP magmatism. Finally, conspicuous by its absence is any evidence for zircon growth or Pb loss between late Archean and recent times. These data indicate there is no evidence for large-scale magmatic or metamorphic events recorded in these xenoliths from 2.5 Ga until the present.
Earthscope could play an important role by facilitating studies that would provide important constraints on the age and composition of the crust in the Northern Rocky Mountains. This information, in turn, could then be integrated with large-scale geophysical studies to improve our broad understanding of the formation and evolution of the North American continent.
Figure 1. Cathodoluminescence images of granulitic zircons from SRP xenoliths. Each of these images is approximately 300 nanometers across. Clockwise from top left: DM-103, COM-9A, COM-22, and SK-3. The grains shown above from DM-103 and COM-9A (top row) yield late Archean ages of 2.58 and 2.77 Ga, respectively, whereas the grains from COM-22 and SK-3 (bottom row) have cores of Archean age and recent (few m.y.) overgrowths. Three 30 nanometer spot analyses (not shown) in the core of SK-3 from left to right yield ages of 2.79, 2.95, and 3.26 Ga, illustrating the complex Archean magmatic/metamorphic history preserved in these grains.
Structure of the Belt Basin: A tentative hypothesis for consideration
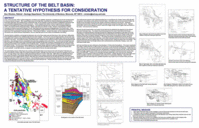
download poster file