X-Ray Fluorescence (XRF)
Karl Wirth, Macalester College and Andy Barth, Indiana University~Purdue University, Indianapolis
What is X-Ray Fluorescence (XRF)
An X-ray fluorescence (XRF) spectrometer is an x-ray instrument used for routine, relatively non-destructive chemical analyses of rocks, minerals, sediments and fluids. It works on wavelength-dispersive spectroscopic principles that are similar to an electron microprobe (EPMA). However, an XRF cannot generally make analyses at the small spot sizes typical of EPMA work (2-5 microns), so it is typically used for bulk analyses of larger fractions of geological materials. The relative ease and low cost of sample preparation, and the stability and ease of use of x-ray spectrometers make this one of the most widely used methods for analysis of major and trace elements in rocks, minerals, and sediment.
Fundamental Principles of X-Ray Fluorescence (XRF)
The XRF method depends on fundamental principles that are common to several other instrumental methods involving interactions between electron beams and x-rays with samples, including: X-ray spectroscopy (e.g., SEM - EDS), X-ray diffraction (XRD), and wavelength dispersive spectroscopy (microprobe WDS).
The analysis of major and trace elements in geological materials by x-ray fluorescence is made possible by the behavior of atoms when they interact with radiation. When materials are excited with high-energy, short wavelength radiation (e.g., X-rays), they can become ionized. If the energy of the radiation is sufficient to dislodge a tightly-held inner electron, the atom becomes unstable and an outer electron replaces the missing inner electron. When this happens, energy is released due to the decreased binding energy of the inner electron orbital compared with an outer one. The emitted radiation is of lower energy than the primary incident X-rays and is termed fluorescent radiation. Because the energy of the emitted photon is characteristic of a transition between specific electron orbitals in a particular element, the resulting fluorescent X-rays can be used to detect the abundances of elements that are present in the sample.
The analysis of major and trace elements in geological materials by x-ray fluorescence is made possible by the behavior of atoms when they interact with radiation. When materials are excited with high-energy, short wavelength radiation (e.g., X-rays), they can become ionized. If the energy of the radiation is sufficient to dislodge a tightly-held inner electron, the atom becomes unstable and an outer electron replaces the missing inner electron. When this happens, energy is released due to the decreased binding energy of the inner electron orbital compared with an outer one. The emitted radiation is of lower energy than the primary incident X-rays and is termed fluorescent radiation. Because the energy of the emitted photon is characteristic of a transition between specific electron orbitals in a particular element, the resulting fluorescent X-rays can be used to detect the abundances of elements that are present in the sample.
X-Ray Fluorescence (XRF) Instrumentation - How Does It Work?
The analysis of major and trace elements in geological materials by XRF is made possible by the behavior of atoms when they interact with X-radiation. An XRF spectrometer works because if a sample is illuminated by an intense X-ray beam, known as the incident beam, some of the energy is scattered, but some is also absorbed within the sample in a manner that depends on its chemistry. The incident X-ray beam is typically produced from a Rh target, although W, Mo, Cr and others can also be used, depending on the application.
When this primary X-ray beam illuminates the sample, it is said to be excited. The excited sample in turn emits X-rays along a spectrum of wavelengths characteristic of the types of atoms present in the sample. How does this happen? The atoms in the sample absorb X-ray energy by ionizing, ejecting electrons from the lower (usually K and L) energy levels. The ejected electrons are replaced by electrons from an outer, higher energy orbital. When this happens, energy is released due to the decreased binding energy of the inner electron orbital compared with an outer one. This energy release is in the form of emission of characteristic X-rays indicating the type of atom present. If a sample has many elements present, as is typical for most minerals and rocks, the use of a Wavelength Dispersive Spectrometer much like that in an EPMA allows the separation of a complex emitted X-ray spectrum into characteristic wavelengths for each element present. Various types of detectors (gas flow proportional and scintillation) are used to measure the intensity of the emitted beam. The flow counter is commonly utilized for measuring long wavelength (>0.15 nm) X-rays that are typical of K spectra from elements lighter than Zn. The scintillation detector is commonly used to analyze shorter wavelengths in the X-ray spectrum (K spectra of element from Nb to I; L spectra of Th and U). X-rays of intermediate wavelength (K spectra produced from Zn to Zr and L spectra from Ba and the rare earth elements) are generally measured by using both detectors in tandem. The intensity of the energy measured by these detectors is proportional to the abundance of the element in the sample. The exact value of this proportionality for each element is derived by comparison to mineral or rock standards whose composition is known from prior analyses by other techniques.
When this primary X-ray beam illuminates the sample, it is said to be excited. The excited sample in turn emits X-rays along a spectrum of wavelengths characteristic of the types of atoms present in the sample. How does this happen? The atoms in the sample absorb X-ray energy by ionizing, ejecting electrons from the lower (usually K and L) energy levels. The ejected electrons are replaced by electrons from an outer, higher energy orbital. When this happens, energy is released due to the decreased binding energy of the inner electron orbital compared with an outer one. This energy release is in the form of emission of characteristic X-rays indicating the type of atom present. If a sample has many elements present, as is typical for most minerals and rocks, the use of a Wavelength Dispersive Spectrometer much like that in an EPMA allows the separation of a complex emitted X-ray spectrum into characteristic wavelengths for each element present. Various types of detectors (gas flow proportional and scintillation) are used to measure the intensity of the emitted beam. The flow counter is commonly utilized for measuring long wavelength (>0.15 nm) X-rays that are typical of K spectra from elements lighter than Zn. The scintillation detector is commonly used to analyze shorter wavelengths in the X-ray spectrum (K spectra of element from Nb to I; L spectra of Th and U). X-rays of intermediate wavelength (K spectra produced from Zn to Zr and L spectra from Ba and the rare earth elements) are generally measured by using both detectors in tandem. The intensity of the energy measured by these detectors is proportional to the abundance of the element in the sample. The exact value of this proportionality for each element is derived by comparison to mineral or rock standards whose composition is known from prior analyses by other techniques.
Applications
X-Ray fluorescence is used in a wide range of applications, including
- research in igneous, sedimentary, and metamorphic petrology
- soil surveys
- mining (e.g., measuring the grade of ore)
- cement production
- ceramic and glass manufacturing
- metallurgy (e.g., quality control)
- environmental studies (e.g., analyses of particulate matter on air filters)
- petroleum industry (e.g., sulfur content of crude oils and petroleum products)
- field analysis in geological and environmental studies (using portable, hand-held XRF spectrometers)
- bulk chemical analyses of major elements (Si, Ti, Al, Fe, Mn, Mg, Ca, Na, K, P) in rock and sediment
- bulk chemical analyses of trace elements (in abundances >1 ppm; Ba, Ce, Co, Cr, Cu, Ga, La, Nb, Ni, Rb, Sc, Sr, Rh, U, V, Y, Zr, Zn) in rock and sediment - detection limits for trace elements are typically on the order of a few parts per million
- relatively large samples, typically > 1 gram
- materials that can be prepared in powder form and effectively homogenized
- materials for which compositionally similar, well-characterized standards are available
- materials containing high abundances of elements for which absorption and fluorescence effects are reasonably well understood
Strengths and Limitations of X-Ray Fluorescence (XRF)?
Strengths
X-Ray fluorescence is particularly well-suited for investigations that involve:
- bulk chemical analyses of major elements (Si, Ti, Al, Fe, Mn, Mg, Ca, Na, K, P) in rock and sediment
- bulk chemical analyses of trace elements (>1 ppm; Ba, Ce, Co, Cr, Cu, Ga, La, Nb, Ni, Rb, Sc, Sr, Rh, U, V, Y, Zr, Zn) in rock and sediment
Limitations
In theory the XRF has the ability to detect X-ray emission from virtually all elements, depending on the wavelength and intensity of incident x-rays. However...
- In practice, most commercially available instruments are very limited in their ability to precisely and accurately measure the abundances of elements with Z<11 in most natural earth materials.
- XRF analyses cannot distinguish variations among isotopes of an element, so these analyses are routinely done with other instruments (see TIMS and SIMS).
- XRF analyses cannot distinguish ions of the same element in different valence states, so these analyses of rocks and minerals are done with techniques such as wet chemical analysis or Mossbauer spectroscopy.
User's Guide - Sample Collection and Preparation
Virtually any solid or liquid material can be analyzed, if adequate standards are available. For rocks and minerals, typical commercial instruments require a sample constituting at least several grams of material, although the sample collected may be much larger. For XRF chemical analyses of rocks, samples are collected that are several times larger than the largest size grain or particle in the rock. This initial sample then suffers a series of crushing steps to reduce it to an average grain size of a few millimeters to a centimeter, when it can be reduced by splitting to a small representative sample of a few tens to hundreds of grams. This small sample split is then ground into a fine powder by any of a variety of techniques to create the XRF sample. Care must be taken particularly at this step to be aware of the composition of the crushing implements, which will inevitably contaminate the sample to some extent.
Data Collection, Results and Presentation
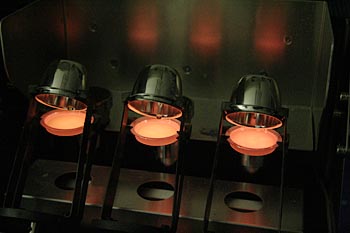
- X-Ray spectrum
- Data table
- Detection limits
- Precision
- Accuracy
- Database and Plotting
- Evaluation of Data Quality (flyers, trends, discriminant fields)
- Geochemical Plots
Literature
The following literature can be used to further explore X-Ray Fluorescence (XRF)
The following literature can be used to further explore the XRF technique and uses of XRF data.
- Fitton, G., 1997, X-Ray fluorescence spectrometry, in Gill, R. (ed.), Modern Analytical Geochemistry: An Introduction to Quantitative Chemical Analysis for Earth, Environmental and Material Scientists: Addison Wesley Longman, UK.
- Potts, P.J., 1987, A Handbook of Silicate Rock Analysis: Chapman and Hall.
- Rollinson, H., 1993, Using Geochemical Data: Evaluation, Presentation, Interpretation: John Wiley, NY.
Related Links
For more information about X-Ray Fluorescence (XRF) follow the links below.
General information about XRF principles and applications:
For more information about XRF laboratories follow the links below:
- LearnXRF.com -- very useful information on principles, instruments and applications (and worthwhile to log-in as an instructor)
- Introduction to XRF -- from Bruker AXS
- Analysis of Rocks Using X-ray Fluorescence Spectroscopy -- a short paper by Timothy LaTour, Georgia State University that presents the basics
- Tutorial on XRF -- a very useful guide to XRF from HORIBA Jobin Yvon, including links to related resources, application notes, and with information on micro-XRF
For more information about XRF laboratories follow the links below:
- Berkeley Archeological XRF laboratory
- University of Massachusetts laboratory
- Michigan State University laboratory
- Washington State University laboratory
- Certified Reference Materials (USGS, GeoStandards)
- International Association of Geoanalysts
- National Institute of Standards and Technology
- Canadian Certified Reference Materials Project
- Geological Survey of Japan
Sources of Geochemical Data
- EarthChem - an international database that combines the assets of PetDB, NavDat, and GeoRoc. Search the database for analyses according to location, rock type, chemical parameters, or references in the literature.
- Geochemical Earth Reference Model (GERM) contains several databases, geochemistry of all reservoirs in the Earth, modern paleo, rock and geomagnetic data
Teaching Activities and Resources
Teaching activities, labs, and resources pertaining to X-Ray Fluorescence (XRF).
For examples of the use of XRF data in teaching activities, check out:
- Geoscience Education in the New Cyberinfrastructure by Kent Ratajeski, Montana State University. These modules provide tutorials and examples covering a range of applications of geologic interest using the EarthChem Geochemical Database. Modules include:
- Physical and Chemical Variations Along the Central American Volcanic Arc by Kent Ratajeski, University of West Georgia
- Global Geochemistry of Mid-Ocean Ridge Basalts by Kent Ratajeski, University of West Georgia
- Volcanic Fields of North America by Kent Ratajeski and Brian Stogner, University of West Georgia
- Volcanic Landforms and Magma Composition by Kent Ratajeski, University of West Georgia
- Cenozoic Volcanic History of the Western United States by Kent Ratajeski, University of West Georgia
- Sr Isotopic Compositions of Mafic Volcanic Rocks, Western United States by Kent Ratajeski, University of West Georgia
- Igneous Rock Compositions and Plate Tectonics by Allen Glazner, University of North Carolina
- Crystallization-Differentiation of Basaltic Magma (Kilauea Iki) by Kent Ratajeski, Montana State University
- Compositional Diversity in Volcanic Suites -- a comparison of rocks of the Mt. Mazama and Yellowstone calderas, by Kent Ratajeski, Montana State University
- Iceland-- a problem set using Excel spreadsheets on Variation Diagrams and the Evolution of Thingmuli Lavas, Iceland by Jeff Tepper University of Puget Sound
- Palisades Sill -- a problem set combining petrography and whole-rock geochemistry on Chemical Differentiation in the Palisades Sill by Matthew Gorring Montclair State University, Dept. of Earth & Env. Studies
- Sierra Nevada Batholith-- an integrated exercise using hand samples, petrography, maps, and whole-rock geochemistry on Magma Modification in the central Sierra Nevada Batholith by Jennifer Wenner University of Wisconsin Oshkosh
Other Resources for Teaching Whole Rock Geochemistry (major and trace elements)
- Trace Elements in Igneous Processes-- PDF with references and problem sets file from Cornell University
- Analysis of Trace Elements in Water--from the USGS
- Understanding Our Planet Through Chemistry-- This U.S.Geological Survey site shows how chemists and geologists use analytical chemistry to: determine the age of the Earth; show an extraterrestrial body collided with the Earth; predict volcanic eruptions; observe atmospheric change over millions of years; and document damage by acid rain and pollution of the Earth's surface.
- Calculation of CIPW Norm from a Bulk Chemical Analsyis-- excel spreadsheet program, used to calculate norm from a bulk chemical analysis, with explanation of "norm" and calculation process